Ultrahigh-pressure liquid chromatography (UHPLC) has been applied successfully to separations of many small molecules, including peptides. Few studies have involved proteins, and these were narrowly focused on the behavior of several purified standards in reversed-phase chromatography (RPC). For this study, the separation of proteins in complex mixtures by ion exchange chromatography (IEX), mixed-bed columns in the case of cell lysates, and cation exchange in the case of hemolyzates was investigated. While most UHPLC studies generate high backpressures as a consequence of using small-diameter particles as the stationary phase, conventional 3- or 5-µm materials at flow rates 4–6 times faster than usual were used. This combination is termed ultrafast flow liquid chromatography (UFFLC). These flow rates generated backpressures of ~5000–7000 psi. Because this is at the top of the range of a conventional HPLC system, a UHPLC system capable of fast flow rates was used. UFFLC is a gradient technique. In the isocratic separation mode, the resolution equation shows significant losses in resolution as the flow rate increases.
Materials and methods
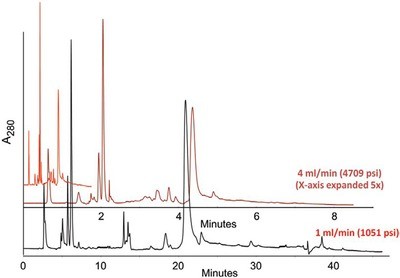
Figure 1 - Effect of flow rate on yeast lysate proteins. Sample: yeast lysate. Column: mixed-bed column—200 × 4.6 mm. Mobile phases—A: 20 mM HEPES, pH 7.0; B: same + 0.8 M NaCl. Black trace: 1 mL/min. Gradient: 0–50%B in 30 min, then 50–100%B in 10 min. Red trace: 4 mL/min. Gradient: 0–8%B in 2 min, then 8–100%B in 5 min. The fast flow trace (red) was elongated along the X-axis (dark red) to permit comparison of the peak shapes and separations. These appear not to have changed significantly even though the running time was 4–5 times faster.
Table 1 - Cost comparison—UFFLC vs current separation methods

Figure 2 - Effect of gradient slope. Sample: yeast lysate. Column: mixed-bed IEX column from Figure 1. Mobile phases—A: 20 mM HEPES, pH 6.6; B: same + 0.8 M NaCl. Flow rate: 4 mL/min. a) Gradient time to 100%B as noted; b) same chromatograms elongated to facilitate comparison; c) same as (b), elongated along the Y-axis to facilitate comparison of minor peaks. (Note: It is generally accepted in chromatography that shallower gradients afford better separations. That was not the case here with either the major or minor protein peaks. The results from the combination of fast flow plus fast gradient are more than acceptable.)
The UFFLC system was a model SYS0201 (Scientific Systems Inc., State College, PA) consisting of two pumps—a model 525 UV-VIS detector and an automatic sample injector. Control and data collection were via EZStart® (Agilent Technologies, Santa Clara, CA). All ion exchange stationary phases were from PolyLC Inc. (Columbia, MD). The mixed-bed column contained PolyCAT A™ (a weak cation exchange material) and Poly-WAX LP™ (a weak anion exchange material) in a 1:1 ratio. Reversed-phase columns were supplied by Optimize Technologies (Oregon City, OR). Column dimensions, pore size, and particle diameter were as indicated. Hemolyzates were a gift from Beverly Vispo (Texas Children’s Hospital, Houston, TX) and were kept frozen until just before analysis. Lysates of yeast cells were a gift from Pierre Havugimana (Dept. of Molecular Genetics, University of Toronto, Ontario, Canada). Mobile phase salts were HPLC grade if available; otherwise, puriss.-grade from Fluka (Buchs, Switzerland) was used.
Experimental results
The analysis of the hemolyzates and lysates clearly demonstrates the resolution and time savings possible with the UFFLC technique. Figure 1 shows the separation of a yeast lysate at 1 mL/min and 4 mL/min. The original separation was done on a conventional gel-type ion exchange resin and the analysis was 120 min with a 30-min regeneration time. The traditional flow rate used to perform these separations is 1 mL/min, the analysis time is typically 40 min, and the equilibration time is typically 20 min, for a total run-to-run analysis of 60 min. At the UFFLC speed of 4 mL/min, the 7-min analysis and 3-min equilibration time add up to a total time of 10 min.
Table 1 shows that the cost savings realized with UFFLC is $73,349 per 1000 samples compared to standard HPLC, and nearly $225,000 per 1000 samples compared to conventional ion exchange methods. This savings is based on the total cost of operation of a small, independent testing laboratory, including prorated equipment costs. The higher flow rate of the UFFLC analysis did not increase the solvent cost. In fact, due to the shorter analysis and equilibration time, the solvent cost was lower than the other methods. The test was performed using the same column size to give an indication of direct scaleup to higher flow. To further reduce the cost, a smaller-diameter column—2 or 3 mm × 25 cm—could have been used at 1 mL/min with the same analysis time and even greater solvent savings.
The effects of gradient slope are shown in Figure 2a and b. Although the longer gradient should provide higher resolution and resolution of more peaks, as shown in the chromatograms, separation is actually better on the fast run. There are more peaks and they are better resolved in the UFFLC run (Figure 2c). This trend can be seen even when smaller columns and particle sizes are used. It is believed that the fast linear velocities and higher number of column volumes per unit time give the proteins less time for isocratic interactions with the column due to the complex multifunctional nature of proteins.