An analytical method for detecting nitrosamines was demonstrated in various water matrices: finished drinking, surface, and treated wastewater effluent. The method uses automated solid-phase extraction (ASPE) followed by analysis with GC-MS/MS using positive chemical ionization (PCI); quantitation was performed using isotope dilution. N-nitrosodimethylamine (NDMA) occurrence was evaluated in effluent from an Australian facility over a three-month period and ranged from 11 to 14 ng/L. This was shown to be a useful and simple analytical method for monitoring nitrosamines.
Background: Nitrosamine formation and GC-MS/MS method development
Nitrosamines have been reported in various matrices.1–7 Formation occurs when precursors8,9 are disinfected1,8,10–15 and are considered by-products. Detection in drinking water is of concern because nitrosamines are probable human carcinogens.16
The U.S. EPA has added five nitrosamines to the Contaminant Candidate List 3 (CCL 3).17 The California Department of Public Health (CDPH) has set Public Health Goals (PHG) and California Notification Levels (CNL).18
A variety of extraction2,9,14,19–27 and analytical methods have been developed.3,14,20,22–26,28–33 The method described here is similar to U.S. EPA Method 52128 with modifications to improve sample throughput, precision, and accuracy. It was evaluated in secondary and tertiary wastewater and expanded to include nine nitrosamines. Analytes were quantified using isotope dilution with labeled standards for each compound, except N-nitrosomethylethylamine (NMEA), which was quantified using NDMA-d6 with good results. A preservative agent was selected to prevent possible microbial degradation in addition to a dechlorinating agent. A series of validation tests were performed to demonstrate the robustness of the method.
Methods and materials
Chemicals, reagents, and samples
Methanol and dichloromethane (DCM) were obtained from Burdick and Jackson (Muskegon, MI). Sodium azide was purchased from Fisher Chemicals, a division of Fisher Scientific (Fair Lawn, NJ), and sodium thiosulfate was from EM Science (Merck KGaA, Darmstadt, Germany). Reagent-grade water was prepared with a Milli-Q gradient water purification system (Millipore, Billerica, MA). Nitrosamine standards were purchased from Ultra Scientific (Kingstown, RI), and isotopically labeled nitrosamines were purchased from Cambridge Isotope Laboratories (Andover, MA). Calibration standards were made in DCM and were replaced every three months. A minimum of seven calibration standards were made, ranging from 1.0 to 250 μg/L.
Tertiary treated effluent was collected downstream from wastewater facilities located in southern Nevada. Raw and finished drinking water collected from a facility in southern Nevada. This method was used to evaluate NDMA occurrence from an Australian advanced wastewater treatment facility. Effluent was sampled four times within a three-month period with additional samples collected for matrix spike and duplicate analysis.
Extraction method
Automated solid-phase extraction was performed using an AutoTrace® workstation Dionex Corp., Sunnyvale, CA). Samples (1000 mL) were processed in batches of six and spiked with isotopically labeled standards prior to extraction. Prepacked activated coconut charcoal cartridges (Resprep™ 521, Restek, Bellefonte, PA) were sequentially conditioned with 5 mL DCM, 5 mL methanol, and 10 mL reagent water. Sample load rate was 15 mL/min. Cartridges were rinsed with 5 mL water and dried for 10 min with nitrogen gas. Analytes were eluted with 10 mL DCM at a flow rate of 5 mL/min. Residual water was removed using sodium sulfate cartridges (Sep-Pak® Dry, Waters Corp., Milford, MA). Extracts were concentrated to a final volume of 500 μL, resulting in a 1:2000 concentration factor.
A CP-3800 gas chromatograph with CP-8400 autosampler was used for all analyses (Varian, Walnut Creek, CA). The injector (Varian 1177) was operated in splitless mode with a Siltek™ deactivated glass liner (Restek) and set at 200 °C. Analytes were separated on a 30 m × 0.32 mm i.d. × 1.8 μm DB 624 column (Agilent, Palo Alto, CA) using a 1.2 mL/min helium flow with an initial pressure pulse of 35 psi for 0.85 min. The temperature program was as follows: 35 °C, hold for 1.0 min; 35–120 °C at 5 °C/min; 120–145 °C at 3 °C/min; 145–250 °C at 35 °C/min, hold for 4.64 min. An injection volume of 2 μL was used for all analyses. The transfer line was set at 250 °C. An example chromatogram of a merged total ion chromatogram (TIC) of 100 μg/L standard is shown in Figure 1.
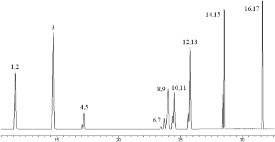
Figure 1 - Merged TIC of 100 μg/L nitrosamine standard. 1) NDMA-d6, 2) NDMA, 3) NMEA, 4) NDEAd10, 5) NDEA, 6) NDPA-d14, 7) NDPA, 8) NMOR-d8, 9) NMOR, 10) NPYR-d8, 11) NPYR, 12) NPIP-d10, 13) NPIP, 14) NDBA-d18, 15) NDBA, 16) NDPhA-d6, 17) NDPhA. For key, see Table 1.
Analysis was performed using a Varian 2200 ion trap mass spectrometer and multiple reaction monitoring (MRM) in positive chemical ionization mode using liquid methanol. Precursor and product ions used for quantitation and confirmation are listed in Table 1 for all nine nitrosamines and corresponding excitation storage and amplitude values. All nitrosamines were analyzed using resonant waveform. Some of the nitrosamines did not exhibit a second product ion in high enough abundance to monitor as a confirmation transition, and therefore have only one quantitation transition. Due to thermal degradation upon injection, N-nitrosodiphenylamine was analyzed as diphenylamine.
Method validation procedure
A method detection limit (MDL) study for NDMA was performed using reagent water fortified with 2.5 ng/L NDMA. The appropriate student’s t-value, for n–1 degrees of freedom, was multiplied by the standard deviation of the replicate samples to calculate the MDL. Method reporting limits (MRLs) (Table 2) were set at three times the MDL for NDMA and greater than 10 times the signal to noise (S/N) for all other nitrosamines.
Spike recovery tests (Table 3) were performed in reagent (n = 5), finished drinking (n = 6), raw (n = 6) (NDMA only), and tertiary wastewater (n = 6) matrices. These were spiked at 12.5 ng/L nitrosamines and labeled standards and quantified using isotope dilution.
Table 1 - Molecular weights and instrument MRM parameters
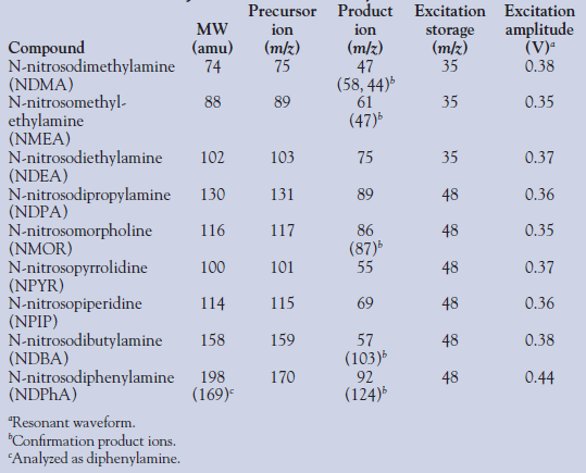
A holding study was prepared with a nonspiked aliquot and duplicate spiked aliquots to form a batch. Each matrix was prepared with preservative/quenching agent batch and another without. The batches were held (4 °C) and then extracted at days 0, 14, and 28, as shown in Table 4.
Table 2 - Nitrosamine reporting limits, CAS numbers, structures, and isotope standards
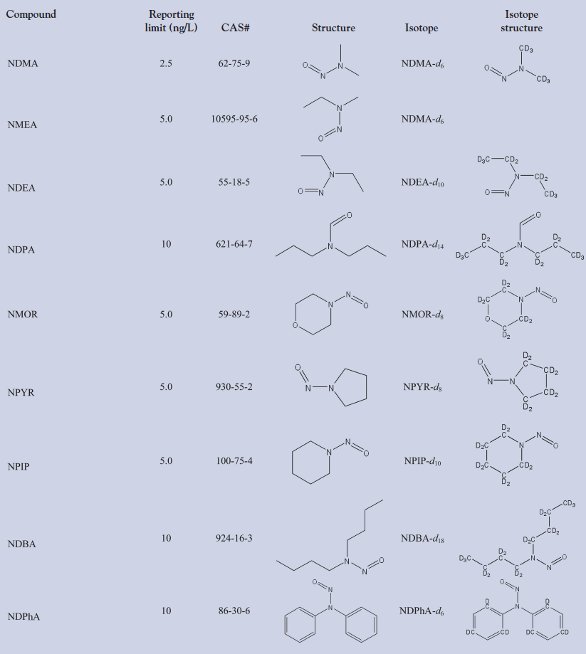
Results and discussion
Method development
Table 3 shows the spike recovery of NDMA ranging from 6 to 12% RSD in the matrices tested. The average recovery for the additional nitrosamines ranged from 91 to 126%, except NDPA in tertiary wastewater at 146%. The method demonstrated good reproducibility, with RSDs below 20% and most less than 10% RSD. The results for nonspiked samples were all less than the MRL, except NMOR, which was detected at an average of 6.1 ng/L in the nonspiked tertiary wastewater. Precision and analyte recovery were demonstrated in all matrices.
Table 3 - Spike recoveries of nitrosamines
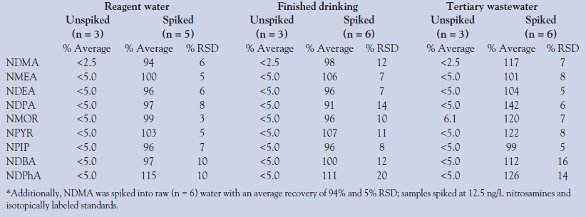
Recoveries for preservative tests (Table 4) were between 96 and 117% in both matrices, for a 28-day holding time, except NDPhA, which had an observable loss by 14 days, indicating that loss was not a function of preservation alone. Recovery in both matrices held for 14 days was 97–127% in preserved samples, but ranged from 72 to 129% in unpreserved matrices. All nonspiked samples were less than the MRL, demonstrating that contamination of NDMA from stock preservatives or a water system was undetectable. Due to the observable loss of NDPhA, a hold time of 14 days was selected and is similar to other methods. Longer hold times were achievable for the other nitrosamines.
Varying cartridge dry times were explored, including 5, 10, and 20 min, in an attempt to remove excess water. A time of 10 min was selected because most of the excess water was removed with minimal loss of analyte. Recoveries diminished with longer drying times. Sodium sulfate cartridges were used to remove remaining water residual.
Automated flow rates and solvent switching systems are more consistent than manual systems. The automated programming provides a batch run time of 1 hr, 45 min. ASPE achieves a high degree of sample throughput and maintains reproducibility.
Table 4 - Average recovery in reagent and raw water with and without preservative and quenching agents (n = 2)
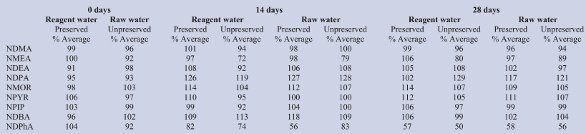
Secondary wastewater effluent samples
NDMA occurrence in secondary wastewater effluent from an Australian facility was evaluated. Concentrations in four samples ranged from 11 to 14 ng/L. Two matrix samples were spiked with NDMA and analyte recovery was 112% and 104%, respectively. Figure 2 is an overlay of chromatograms from a wastewater extract and 2.5 μg/L and 10 μg/L NDMA standards.
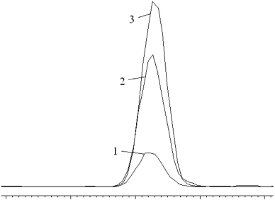
Figure 2 - Overlapping 47 m/z extracted ion chromatogram (XIC) of 1) 2.5 μg/L NDMA standards, 2) 10 μg/L NDMA standards, and 3) extracted secondary treated wastewater effluent.
Conclusion
Automated solid-phase extraction is beneficial for laboratories that monitor nitrosamines on a routine basis and in many water matrices. The technique demonstrates improved sample throughput over traditional methods of extraction. An advantage of automating the SPE is reduction of errors and variability due to sample handling during intricate extraction steps and flow rate consistency. The recent availability of isotopically labeled standards provides the advantage of using isotope dilution for quantification of each compound, while correcting for ASPE loss and method variability. Laboratories can apply this simple and robust method to evaluate nitrosamine occurrence in a variety of water matrices with improved sample throughput and achieve sensitivity comparable to U.S. EPA Method 521 and others.
References
- Pehlivanoglu-Mantas, E.; Hawley, E. et al. Formation of nitrosodimethylamine (NDMA) during chlorine disinfection of wastewater effluents prior to use in irrigation systems. Water Res. 2006, 40, 341–7.
- Barrett, S.; Hwang, C. et al. Occurrence of NDMA in drinking water: a North American Survey, 2001–2002. In American Water Works Association Annual Conference: Anaheim, CA, 2003, pp 1–19, American Water Works Association.
- Planas, C.; Palacios, O. et al. Analysis of nitrosamines in water by automated SPE and isotope dilution GC/HRMS occurrence in the different steps of a drinking water treatment plant, and in chlorinated samples from a reservoir and a sewage treatment plant effluent. Talanta2008, 76(4), 906–13.
- Yurchenko, S.; Molder, U. Volatile N-nitrosamines in various fish products. Food Chemistry2006, 96, 325–33.
- Reinik, M.; Tamme, T. et al. Nitrites, nitrates and N-nitrosoamines in Estonian cured meat products: intake by Estonian children and adolescents. Food Addit. Contam. 2005, 22(11), 1098–105.
- Shin, J.H.; Chung, M.J. et al. Occurrence of N-nitrosodimethylamine in South Korean and imported alcoholic beverages. Food Addit. Contam. 2005, 22(11), 1083–6.
- Bradley, P.; Carr, S. et al. Biodegradation of N-nitrosodimethylamine in soil from a water reclamation facility. Bioremediation J. 2005, 9(2), 115–20.
- Siddiqui, M.; Atasi, K.Z. N-nitrosodimethylamine: a disinfectant byproduct and its occurrence in wastewater. Water Environ. Res. 2004, 76(4), 316–26.
- Mitch, W.A.; Sedlak, D.L. Characterization and fate of N-nitrosodimethylamine precursors in municipal wastewater treatment plants. Environ. Sci. Technol. 2004, 38(5), 1445–54.
- Wilczak, A.; Lai, H. et al. NDMA formation in chloraminated distribution system: is there an effect of nitrification?—EMBUD experience. In American Water Works Association Annual Conference: Orlando, FL, 2004, pp 1–9, American Water Works Association.
- Mitch, W.A.; Sedlak, D.L. Formation of N-nitrosodimethylamine (NDMA) from dimethylamine during chlorination. Environ. Sci. Technol. 2002, 36(4), 588–95.
- Mitch, W.A.; Sharp, J. et al. N-nitrosodimethylamine (NDMA) as a drinking water contaminant: a review. Environ. Eng. Sci. 2003, 20(5), 389–404.
- Pehlivanoglu-Mantas, E.; Sedlak, D.L. The fate of wastewater-derived NDMA precursors in the aquatic environment. Water Res. 2006, 40, 1287–93.
- Brubacher, C.; Andrews, S. et al. NDMA formation from wastewater treatment processes for water reuse: stage 1 results. In American Water Works Association Annual Conference: Anaheim, CA, 2003, pp 1–11, American Water Works Association.
- Andrzejewski, P.; Kasprzyk-Hordern, B. et al. The hazard of N-nitrosodimethylamine (NDMA) formation during water disinfection with strong oxidants. Desalination2005, 176, 37–45.
- Guidelines for Drinking-Water Quality, 3rd ed., including 1st and 2nd addenda, WHO, 2008.
- Contaminant Candidate List 3. 2009 [cited 2010 02/03/2010]; http://water.epa.gov/ scitech/drinkingwater/dws/ccl/ccl3.cfm#ccl3.
- NDMA and Other Nitrosamines—Drinking Water Issues. 2009 [cited 02/16/2010]; http://www.cdph.ca.gov/certlic/drinkingwater/Pages/NDMA.aspx.
- Cheng, R.; Andrews-Tate, C. et al. Development of low cost method(s) for NDMA analysis: round-robin testing. In American Water Works Association Water Quality Technology Conference: San Antonio, TX, 2004, pp 1–20, American Water Works Association.
- Plumlee, M.H.; López-Mesasa, M. et al. N-nitrosodimethylamine (NDMA) removal by reverse osmosis and UV treatment and analysis via LC–MS/MS. Water Res. 2008, 42(1–2), 347–55.
- Andrews-Tate, C.; Echarte, M. et al. Optimizing nitrosamine analysis by solid phase extraction. In American Chemical Society Division of Environmental Chemistry: Anaheim, CA, 2004, pp 283–7, American Chemical Society.
- Guo, Y.; Hwang, C. et al. Analysis of trace level NDMA in drinking water. In American Chemical Society Division of Environmental Chemistry: San Diego, CA, 2001, pp 663–7, American Chemical Society.
- Charrois, J.W.; Arend, M.W. et al. Detection of N-nitrosamines in water using solid phase extraction combined with GC/MSD ammonia positive chemical ionization. In American Water Works Association Water Quality Technology Conference: Philadelphia, PA, 2003, pp 1–11, American Water Works Association.
- Fields, J.; Andrews-Tate, C. et al. Optimization of SPE and GC/CI-MS/MS as analytical methods for extraction and detection of NDMA. In American Water Works Association Water Quality Technology Conference: San Antonio, TX, 2004, pp 1–17, American Water Works Association.
- Zhao, Y.Y.; Boyd, J. et al., Characterization of new nitrosamines in drinking water using liquid chromatography tandem mass spectrometry. Environ. Sci. Technol. 2006, 40(24), 7636–41.
- Luo, X.; Clevenger, T. et al. NDMA analytical methods comparisons and its occurrence in Missouri. In American Water Works Association Water Quality Technology Conference: Philadelphia, PA, 2003, pp 1–12, American Water Works Association.
- Tomkins, B.A.; Griest, W.H. Determinations of N-nitrosodimethylamine at part-per-trillion concentrations in contaminated groundwaters and drinking waters featuring carbon-based membrane extraction disks. Anal. Chem. 1996, 68(15), 2533–40.
- Munch, J.W.; Bassett, M.V. Method development for the analysis of N-nitrosodimethylamine and other N-nitrosamines in drinking water at low nanogram/liter concentrations using solidphase extraction and gas chromatography with chemical ionization tandem mass spectrometry. J. AOAC Int. 2006, 89(2), 486–97.
- Schreiber, I.M.; Mitch, W.A. Occurrence and fate of nitrosamines and nitrosamine precursors in wastewater-impacted surface waters using boron as a conservative tracer. Environ. Sci. Technol. 2006, 40(10), 3203–10.
- Charrois, J.W.; Arend, M.W. et al. Detecting N-nitrosamines in drinking water at nanogram per liter levels using ammonia positive chemical ionization. Environ. Sci. Technol. 2004, 38(18), 4835–41.
- Plomley, J.; Koester, C. et al. Determination of N-nitrosodimethylamine in complex environmental matrices by quadrupole ion storage tandem mass spectrometry enhanced by unidirectional ion ejection. Anal. Chem. 1994, 66(24), 4437–43.
- Lee, C.; Schmidt, C. et al. Oxidation of N-nitrosodimethylamine (NDMA) precursors with ozone and chlorine dioxide: kinetics and effect on NDMA formation potential. Environ. Sci. Technol. 2007, 41(6), 2056–63.
- Sharpless, C.M.; Linden, K.G. Experimental and model comparisons of lowand medium-pressure Hg lamps for the direct and H2O2 assisted UV photodegradation of N-nitrosodimethylamine in simulated drinking water. Environ. Sci. Technol. 2003, 37(9), 1933–40.
Janie C. Holady, B.S., is a Chemist, and Rebecca A. Trenholm, M.S., is a Research Chemist, Water Quality Research and Development Division, Southern Nevada Water Authority, P.O. Box 99954, Las Vegas, NV 89193-9954, U.S.A.; tel.: 702- 856-3662; fax: 702-856-3647; e-mail: [email protected]. Shane A. Snyder, Ph.D., is a Professor, Chemical and Environmental Engineering, University of Arizona, Tucson, AZ, U.S.A.