Since the introduction of ultrahigh-performance liquid chromatography (UHPLC) in 2004, one of the most significant technological advancements has been the introduction of core–shell columns. A chromatographic column packed with core–shell particles offers several significant advantages over columns packed with fully porous sub-2-μm particles. This article discusses the advantages and history of core–shell particle development. Considerations for choosing a core–shell column will be given as well.
The concept of superficially porous or, as they are now commonly referred to, core–shell, HPLC sorbents was first introduced over 50 years ago. One of the earliest examples of this concept was the pellicular, or thin-skin, sorbents prepared by Horváth and Lipinski in the late 1960s, consisting of 30–50 μm, nonporous, solid glass spheres coated with a 1–2 μm thick layer of porous organic polymer.1 This layer served as the chromatographically active surface via chemical modifications such as cation and anion exchange functionalities. The major drawbacks of the pellicular sorbents were 1) very limited surface area manifesting itself as low retention and sample loading capacity, and 2) poor uniformity and mechanical stability of the porous layer. Also in the late 1960s, J.J. Kirkland prepared what he called superficially porous sorbents by successively fusing layers of nanometer-sized nonporous inorganic spherical particles (or nanoparticles) to a 10-μm nonporous solid spherical core.2 The network of spaces between the fused nanoparticles formed the chromatographically active pore volume. One of the preferred inorganic substances used to prepare the superficially porous sorbents was silica, which made further chemical modification of the pore surface, e.g., with C18, relatively straightforward.
Advances in column packing materials
The primary drivers for investigating core–shell type sorbents for LC were improving speed while maintaining or improving performance. At the time of these early innovations, it was well understood that higher performance in LC could be achieved with the use of smaller particles. While in the late 1960s and early 1970s the most commonly used sorbent for LC was 10–50 μm irregularly shaped silica, spherical 10-μm particles were developed in the mid-1970s; 5-μm spherical particles were developed in the ’80s. However, the capabilities of both silica manufacturing and LC systems lagged behind the requirements imposed by smaller particles. Therefore, at the time of these advancements (and arguably even today) core–shell type sorbents offered an ideal compromise between the capabilities of silica manufacturing technology and those of the existing liquid chromatographic systems.
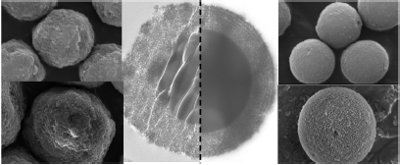
Figure 1 - SEM and TEM images of Halo® (Advanced Material Technologies, Wilmington, DE) (left) and Kinetex® (Phenomenex, Torrance, CA) (right) particles.
Several core–shell type sorbents (also called pellicular sorbents) were commercialized in the late 1970s. While these sorbents offered performance benefits over fully porous sorbents of similar size, the benefits were often outweighed by the very low loading capacities and poor analyte retention consequences of very low surface areas, typically in the range of 5–15 m2/g. This shortcoming is not inherent to the concept, but rather is a consequence of the state of sol–gel chemistry in the 1970s. Parallel advancements in the manufacture of fully porous silica produced smaller and smaller particles, with the latest generation being sub-2 μm in diameter, which were introduced in 2004. The performance achievable with these particles and the instrumentation capable of operating columns made with them initially constituted what is collectively called UHPLC.
Sol–gel technology
The dominance of fully porous sub-2-μm particles was short-lived with the reintroduction of core–shell particles in 2007. This new generation of core–shell particles took advantage of the many advances in silica sol–gel technology, providing increased surface area (typically 100–150 m2/g) and a very narrow particle size distribution. The first commercially available sorbent in this new generation of core–shell particles was the Halo® from Advanced Material Technologies (Wilmington, DE). The Halo particle is 2.7 μm in diameter with a shell thickness of 0.5 μm (see its morphology depicted by scanning electron microscopy [SEM] and transmission electron microscopy [TEM] images in Figure 1). Columns packed with Halo core–shell particles give efficiency values close to those of sub-2-μm fully porous sorbents but at significantly lower operational pressure.
Table 1 - Suppliers of core–shell columns
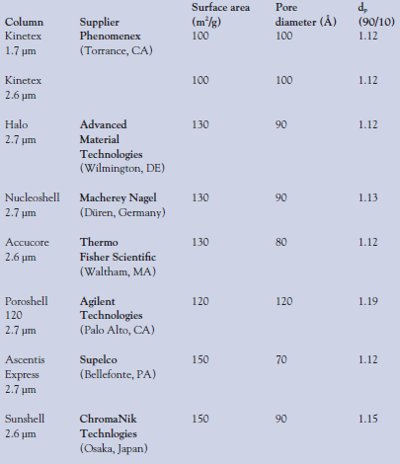
Advanced HPLC instruments and chromatography columns
The rapid growth of UHPLC was facilitated by the introduction of advanced HPLC instrumentation, such as the Agilent 1200 series (Agilent Technologies, Palo Alto, CA) and the ACQUITY UPLC from Waters (Milford, MA), along with high-efficiency chromatographic columns such as 1.8-μm Rapid Resolution sorbents from Agilent and the ACQUITY 1.7-μm line from Waters. Due to the very high operational pressures required to operate sub-2-μm sorbents near their optimal linear velocity, it was incorrectly assumed by many that ultrahigh performance required ultrahigh pressure. The Halo core–shell sorbent demonstrated that the ultrahigh-efficiency characteristic of UHPLC can be achieved at conventional HPLC pressures. Since the introduction of the Halo core–shell sorbent, several similar sorbents have been released. A list of companies offering core–shell sorbents along with the physical properties of each are shown in Table 1.
In 2009, the latest generation of core–shell sorbents was introduced by Phenomenex under the trade name Kinetex in two particle sizes—2.6 μm and 1.7 μm. The major improvement in sorbent architecture in the Kinetex core–shell sorbents is the smoother surface and spherical shape of the particles. An SEM picture of a Kinetex particle and a TEM picture of a cross-section of a Kinetex particle are shown in Figure 1.
It is thought that the physical characteristics of the Kinetex particles are partly responsible for the improved performance when compared to other core–shell and fully porous sorbents. The Kinetex 1.7-μm sorbent is the only commercially available sub-2-μm core–shell particle and provides the highest performance (i.e., the lowest height equivalent to a theoretical plate) available with any UHPLC column.3
Over 150 technical publications to date have demonstrated the performance of core–shell sorbents and numerous others were dedicated to comparing them to fully porous sub-2-μm sorbents. The general consensus in the literature has been that the performance of the former particles is at least as good if not better than sub-2-μm fully porous particles. In order to realize the full performance benefits of core–shell sorbents, particular attention must be paid to extra column volume in the (U)HPLC system. UHPLC performance can be obtained on conventional HPLC systems with core–shell columns of larger volume (e.g., 100 × 3 or 75 × 4.6 mm) provided the HPLC system has been optimized to reduce extra column volume.
Role of the HPLC system in core–shell column performance
When selecting a core–shell column, one of the primary considerations is the HPLC system it will be used with. Even the most current UHPLC systems have limitations regarding how well they can reflect the true performance of a core–shell column. While no simple modifications are available to the practitioner to improve the current performance of UHPLC instruments, judicious selection of the column dimensions can alleviate the situation. While narrow-bore columns are the most susceptible to instrument shortcomings (with the 50 × 2.1 mm column being the most demanding4 since it generates peaks with volumes of only 10–20 μL), a 3.0-mm-i.d. column will be more tolerant of instrument deficiencies (since it produces peaks with volumes approximately two times larger or 20–40 μL). Therefore, the effect of extra column volume will be less pronounced as the volume (both length and i.d.) of the column is increased. Still, if the intended application requires the highest speed, then the most demanding column format, 50 × 2.1 mm, may be required; however, the 50 × 3.0 mm should be considered as an acceptable compromise. One further consideration is the pronounced negative effect column selectors have on column performance due to the significant extra column volume they typically introduce.
Core–shell sorbents are the best-suited sorbent for fast analyses because their optimum linear velocity is significantly higher than that of fully porous sorbents and their exceptional performance is preserved even when operated at linear velocities significantly above the optimum value. Hence, analysis times can be significantly reduced with core–shell columns by increasing flow rate. This consistent performance of core–shell particles across a much wider and higher linear velocity range is explained by their high thermal conductivity, which alleviates the negative impact of frictional heating. 5 This phenomenon is associated with the high operational pressures required in fast analyses with sub-2-μm particles. The high thermal conductivity of the Kinetex 1.7-μm particle ensures effective dissipation of the heat generated by the mobile phase passing through the column packing at very high velocity.
This additional positive characteristic of core–shell particles should be extremely beneficial with future advancements in the field, when even smaller particles requiring even higher operational pressures will be developed. The significant heat generated when using such sorbents for very fast analyses can be effectively dissipated due to their high thermal conductivity. This characteristic positions core–shell sorbents as the media of the future since fully porous particles have been shown to lose significant performance at high flow rates due to frictional heating.
References
- Horváth, C.G.; Preiss, B.A. et al. Anal. Chem.1967, 39, 1422.
- Kirkland, J.J. Superficially Porous Supports for Liquid Chromatography, U.S. Patent 3505785, 1970.
- Fekete, Sz.; Ganzler, K. et al. J. Pharm. Biomed. Anal.2011, 54, 482–90.
- Gritti, F.; Sanchez, C.A. et al. J. Chromatogr. A2010, 1217(18), 3000–12.
- Gritti, F.; Guiochon, G. J. Chromatogr. A2010, 1217(31), 5069–83.
Carl Sanchez, B.Sc., is Senior Research Scientist, and Tivadar Farkas, Ph.D., is Senior Managing Scientist, Phenomenex, 411 Madrid Ave., Torrance, CA 90501-1430, U.S.A.; tel.: 310-212-0555; fax: 310-328-7768; e-mail: [email protected].