This is the fourth in a series of articles entitled, “Minimizing Liquid Delivery Risk,” addressing the most common causes of liquid delivery error and providing guidance on how to address and overcome these errors. This article continues the discussion of errors associated with handheld pipets, focusing on environmental variables in the laboratory, which can lead to significant inaccuracies of volumes delivered by air-displacement pipets.
The first and third article in this series focused on manually operated pipets as sources of error, caused either by mechanical failures1 or induced by poor operator technique.2 Regular calibration and verification cycles to monitor the mechanical performance of pipets, combined with thorough training of pipet operators, are essential to prevent costly errors to any laboratory or institution. Properly devised standard operating procedures (SOPs) can minimize such error sources to a large extent.
Whenever experimental results deviate from the anticipated results, it is common to examine the reagents, experimental protocols, instruments, and even the researchers’ techniques for potential sources of the error. Often, the root cause can be identified this way and subsequently be eliminated.
In light of the multitude of error sources, it is easy to overlook the environmental conditions in the laboratory, and the effects they can exert on the quantitative dispensing of liquid with handheld pipets. This article focuses on only two of these variables: barometric pressure and thermal disequilibrium between reagents and pipets.
Air-displacement and positive-displacement liquid delivery
To understand how these environmental conditions affect the performance and operation of air-displacement pipets, one has to understand the way these instruments operate and how they are calibrated by their manufacturers. In mechanically operated air-displacement pipets, a piston moves inside the pipet shaft, increasing or decreasing the air volume inside of the shaft. The air cushion, which is created inside of the pipet shaft once the pipet tip is immersed in the sample liquid, couples the piston to the liquid and effects the aspiration of the sample into the pipet tip. This mechanism allows the sample to contact only the disposable tip, thus allowing the rapid, successive use of the pipet with different samples by simply changing tips. The construction and operating principle, however, render these pipets susceptible to variations in barometric pressure, temperature, and humidity.
This susceptibility of pipetted volumes to environmental influences is recognized in international pipet calibration standards such as ISO 8655-2, which stipulates that pipets must be adjusted to deliver correctly at 101 kPa barometric pressure (sea level), 50% relative humidity, and 20 °C. Furthermore, the related part six of this standard (ISO 8655-6) requires that equipment (pipet and tips) be brought into thermal equilibrium with the sample solutions by waiting at least two hours prior to performing calibration testing.
These tightly specified conditions can be met in carefully controlled calibration laboratories. The vast majority of scientists, however, often use airdisplacement pipets under conditions that differ significantly from these calibration specifications.
Some liquid delivery instruments use positive displacement rather than air displacement. In these devices, including syringes, certain handheld pipets, and some automated liquid handlers, the piston is in direct contact with the sample to be dispensed. While these devices are largely unaffected by changes in environmental conditions, tedious cleaning procedures and the increased danger of cross-contamination are two disadvantages limiting widespread use.
Effect of barometric pressure
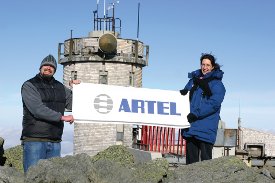
Figure 1 - The ARTEL Extreme Pipetting Expedition trekked to Mount Washington, NH, to study how altitude affects liquid handling accuracy.
In most cases, pipets are calibrated by their manufacturers in laboratories that operate at barometric pressures that correspond to near sea-level altitude. Many laboratories, however, are located at altitudes that are significantly above sea level. As elevation increases, barometric pressure decreases.
The scientists at ARTEL (Westbrook, ME) investigated the impact that differences in barometric pressure exert on the accuracy of pipetted volumes. A set of fixed-volume pipets and a set of adjustable-volume pipets, all from leading manufacturers, were tested at an altitude of 1917 m (6288 ft) on top of Mount Washington in New Hampshire (Figure 1). The results obtained at this elevation were compared to the volumes measured in ARTEL’s accredited laboratory (ISO 17025) under controlled conditions at sea level in coastal Maine. The same pipets were operated by the same technicians to minimize operator variability.
To ensure a scientifically sound comparison of the data, the accuracy and precision of the pipets were determined with the PCS® (Pipet Calibration System, ARTEL). Unaffected by differences in barometric pressure, the PCS acquires NIST-traceable data, based on the principle of ratiometric photometry, regardless of where the data collection takes place.
Table 1- Effect of barometric pressure on fixed-volume pipets; 22 data points were acquired for each volume setting by each operator

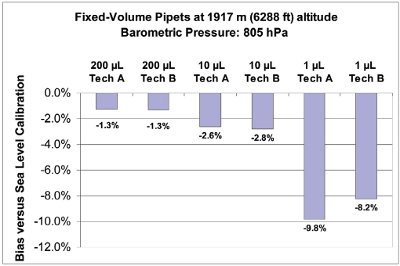
Figure 2 - Inaccuracies of fixed-volume pipets at high altitude normalized to sea-level calibration data in the ARTEL laboratory.
The dispensing accuracy of three fixed-volume micropipets of commonly used volumes (200 μL, 10 μL, and 1 μL) were evaluated by two scientists who were trained and certified using the ARTEL Method™ (a comprehensive, science-based training suite for standardizing and optimizing pipetting skills). The data presented in Table 1 show that all of the pipets underdelivered at high altitude, compared to the sea-level laboratory data. Figure 2 shows the underdelivery of the pipets, after normalizing it to the laboratory data. It is evident that smaller volumes are more affected by the influence of reduced barometric pressure: The 1-μL pipet consistently underdelivered by 9%, while the 200-μL pipet underdelivered by only 1.3%. The data also demonstrate that the variation between both trained and certified operators is minimal.
Table 2 - Inaccuracies in volume delivery by adjustable-volume pipets at 1917 m (6288 ft) and at sea level; 10 data points were acquired for each volume setting

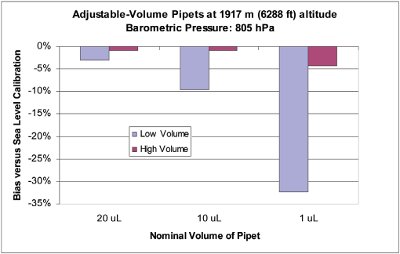
Figure 3 - Adjustable-volume pipets at nominal volume and at 10% of nominal volume settings. Volume delivery inaccuracies are normalized to sea-level calibration data.
The liquid delivery of three adjustable-volume pipets (20 μL, 10 μL, and 2 μL) was investigated at their nominal volume, as well as at 10% of their nominal volume. The data for both conditions, high altitude and sea level, are summarized in Table 2, and the normalized high-altitude data are shown in Figure 3. All tested pipets underdelivered at both volume settings when used under reduced barometric pressure. Concurrent with the fixed-volume pipets, the results show that smaller volumes are more affected by the change in pressure than are larger volumes: While the 2-μL pipet underdelivered by 4.3% at its nominal volume, the 20-μL pipet exhibited underdelivery of less than 1%. Induced inaccuracies were found to be significantly larger when the pipets were used at 10% of their nominal volume, with errors ranging from 3% to over 30% underdelivery.
Table 3 - Liquid delivery by calibrated syringes at 805 hPa of barometric pressure, and at 1917 m (6288 ft) altitude

Volume control samples were dispensed via calibrated glass syringes (200 μL, 40 μL, and 8 μL), which operate by a positive-displacement mechanism and are not sensitive to changes in barometric pressure. These controls yielded an average inaccuracy of 0.52% or less for both technicians, as shown in Table 3. This confirms that the PCS is operating within its specifications and is not affected by the change in barometric pressure due to the change in altitude.