The rheology of a suspension or dispersion relates directly to many parameters of commercial interest. For example, the rheological properties of foodstuffs can be correlated with sensory variables such as “feel in the mouth” and spreadability, while the viscosity of paints must be closely matched to the application method for optimal performance. As a result, developers and manufacturers of a wide range of products need to measure, understand, and control rheological properties.
The rheology of a suspension is influenced by particle size, particle size distribution, and the volume fraction of solids present. Zeta potential, a measure of the charge on particles in the system, also has a marked effect. In this article, the relationships between rheology, especially viscosity, and particle size parameters and zeta potential are examined in detail. The information provided is intended to give both researchers and developers sufficient understanding to successfully manipulate variables in order to produce materials with the required viscosity. Some basic descriptions of commonly used rheological terms are provided, and data from example systems are presented to illustrate key points.
Defining terms
Viscosity, most commonly thought of as “thickness,” is the term used to describe resistance to flow. Liquids with high viscosity are relatively immobile when subjected to shear, i.e., when a force is applied to make them move. Low-viscosity fluids flow relatively easily. Viscosity can be measured easily together with other rheological properties, using either capillary or rotational rheometers, depending on the properties of the material being tested and the nature of the data required.
Shear rate is the term that defines the speed with which a material is being deformed. During some processes, such as spraying, materials are subjected to high shear (>105 s–1); in others, pumping or leveling, for example, the associated shear rate is low (10–1–101 s–1). High shear rates tend to occur when a material is being forced rapidly through a narrow gap.
If viscosity remains constant while shear rate increases, then a fluid is described as being Newtonian. Non-Newtonian fluids, which do not exhibit this behavior, fall into one of two categories—shear thinning or shear thickening. With shear thinning materials, as the name suggests, viscosity decreases with increasing shear rate. As shear is applied, the structure of the material breaks down and it flows more readily. Most fluids and semisolids fall into this group. Conversely, shear-thickening materials exhibit increased viscosities at increasing shear rates.
For suspensions, the volume fraction and the maximum volume fraction can also influence viscosity. Maximum volume fraction (the highest volume of particles that can be added to a fluid) can be thought of as the amount of free space the particles have in which to move around. Its impact on viscosity is discussed below.
The other parameter considered here is zeta potential. Zeta potential is a measure of electrostatic charge at the particle boundary, defined so as to include solvating ions. Zeta potential can therefore only be measured for the system as a whole. A high zeta potential, either positive or negative, is associated with strong repulsive forces between particles. In systems with lower zeta potentials, the repulsive forces are weaker and may be overcome by attractive van der Waals forces.
The following sections discuss the impact on viscosity of particle size, particle size distribution, volume fraction, and zeta potential.
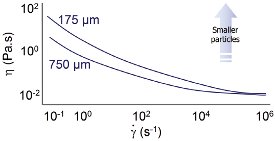
Figure 1 - Impact of particle size on viscosity.
If the total mass of particles in a suspension is kept constant but the particle size of the solid phase is reduced, then the net effect is an increase in the number of particles in the system. The impact on viscosity of such a change, across a range of shear rates, is shown in Figure 1.
The data presented here are for latex particles in a pressure-sensitive adhesive. The first conclusion that can be drawn from the shape of the graph is that the fluid is shear thinning—viscosity decreases at higher shear rates. The second is that viscosity tends to be higher with smaller particles. As noted above, the change in particle size is associated with an increase in particle number if all other system variables are kept constant. Smaller particles therefore result in an increase in the number of particle–particle interactions and an increase in resistance to flow.
It is clear that as shear rate increases, this effect becomes less marked. This suggests that any particle–particle interactions are relatively weak and are broken down at high shear rates.
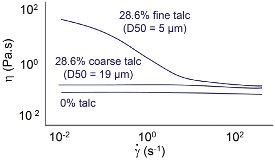
Figure 2 - Impact of particle size on flow behavior.
Data for a talc/epoxy system are shown in Figure 2. The epoxy system is Newtonian in the absence of talc. The addition of coarse talc results in an increase in viscosity, but the system remains Newtonian. The addition of a finer talc results in a further, more significant increase in viscosity, particularly at low shear rates. Colloidal repulsion between a relatively large number of particles gives structure to the fluid, increasing resistance to flow. As in the previous example, this relatively weak structure is broken down at high shear rates. The fluid has become shear thinning.
Volume fraction
The effects of volume fraction and maximum volume fraction on viscosity are described using the Krieger-Dougherty equation:
where η is the viscosity of the suspension; ηmedium is the viscosity of the base medium; Φ is the volume fraction of solids in the suspension; Φm is the maximum volume fraction of solids in the suspension; and [η] in the intrinsic viscosity of the medium, which is 2.5 for spheres.
This correlation indicates that viscosity increases with increasing volume fraction. As the volume fraction of solids in the system increases, the particles become more closely packed together, it becomes more difficult for them to move freely, particle–particle interactions increase, and resistance to flow (viscosity) rises. As the volume fraction nears the maximum that the sample can contain, then viscosity rises very steeply.
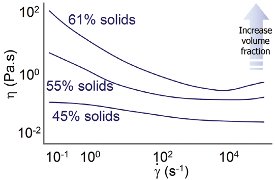
Figure 3 - Viscosity as a function of shear rate for different volume fractions.
As well as influencing the absolute value of viscosity, volume fraction also has an impact on the nature of the relationship between shear rate and viscosity for the system—flow behavior. Suspensions with relatively low volume fraction tend to behave as Newtonian fluids—viscosity is independent of shear rate. As volume fraction is increased, shear-thinning behavior is observed. This transition is illustrated in Figure 3 for a latex/pressure-sensitive adhesive system.
At the lowest volume fraction, the system is almost Newtonian. As volume fraction is increased, shear-thinning behavior becomes evident. Increasing the volume fraction results in a higher degree of particle–particle interaction and resistance to flow increases. The forces between particles are, however, broken down at high shear rates.
A further transition in flow behavior occurs as volume fraction is increased to values above ≈ 50% of maximum volume fraction. At these solids loadings, the free movement of particles is significantly hindered as collisions between particles increase and the system effectively becomes more congested. As shear rate is increased, the particles are trying to move more rapidly and thus the effect becomes more pronounced. Viscosity therefore increases with shear rate; the system is shear thickening at very high shear rates.