Derived from biological sources, biodiesel is gaining momentum as the energy source of the future, being biodegradable and nontoxic, and producing around 60% less net carbon dioxide emissions than petroleum-based diesel. Biodiesel is composed of methyl esters of fatty acids, wheras diesel fuels predominantly contain a mixture of C10 through C19 hydrocarbons, including approximately 64% aliphatic hydrocarbons, 1–2% olefinic hydrocarbons, and 35% aromatic hydrocarbons.
The “B” factor is an international system used to state the amount of biodiesel in any fuel mix. For example, fuel containing 20% biodiesel is labeled B20, and pure biodiesel is referred to as B100. One of the greatest advantages of biodiesel is that it can be used in diesel engines without requiring any significant modifications of the engine, and can be blended at any percentage volume with petroleumbased diesel fuel; the impact of lower emissions is usually proportional to the fraction of biodiesel being used.
The American Society for Testing and Materials (ASTM) has adopted two specifications to regulate the quality of biodiesel (ASTM D 6751) that is to be used in blends up to 20% and the quality of diesel fuel (ASTM D 975). Use of any fuel that does not meet its quality specifications can cause performance problems or equipment damage.
If the biodiesel–diesel fuel blend level is known, changes in engine performance and emissions can be predicted and eliminated, if needed, either by injecting more fuel or by adjusting the fuel injection timing. On the other hand, determining the percentage volume of biodiesel in diesel blends is especially important to specify the purity level of the blend. In order to specify the biodiesel–diesel fuel blend level, scientists must have advanced knowledge of biodiesel fuel properties, their impact on engine performance and emissions, and the blend characteristics of biodiesel.
FT-NIR: A traditional approach
Fourier-transform near-infrared (FT-NIR) spectroscopy is a traditional technique based on a Michelson interferometer. FT-NIR achieves excellent resolution and is capable of providing very high reproducibility of spectra, but is better configured for operating in nonvibrational, laboratory environments. In a process environment, temperature fluctuations and vibrations related to machinery can adversely affect the performance in certain cases of the interferometer and can cause various problems due to the effects of the jitter in the moving mirror.
EP-NIR: An innovative alternative
Encoded photometric near-infrared (EP-NIR) spectroscopy technology has emerged as a more rugged solution than FT-NIR. EP-NIR technology is military certified for vibration insensitive operation under Military 202G Method 204D. A high-frequency vibration resistance test was performed to determine the effect of vibration on component parts of the analyzer in sweeping frequency ranges of 0.5–30 Hz. EP-IR demonstrated no degradation in electrical or photometric performance during or after the test. Moreover, the photometric performance was further tested by collecting spectra as these vibrations were applied to the analyzer. Even under the stress of such vibrations, the EP-NIR analyzer retained photometric performance and met root mean square (RMS) signal-to-noise specifications greater than 50,000:1.
EP-NIR spectroscopy is designed to encode analytical information similar to FT-NIR interferometers but without the environmentally sensitive components of such instruments. EP-NIR covers a wide spectral range of 1375–2750 nm, whereas traditional NIR systems typically stop at 2100 nm. This unique capability makes EP-NIR well suited to a wide range of applications including protein/fat analysis, petrochemicals, biofuels, and alcohols/starch. EP-NIR facilitates fast scanning operation, being capable of simultaneously measuring multiple gas components in the ppb to ppm range at an impressive rate of 100 scans/sec. This results in high sample throughput, real-time quality control monitoring, and a high degree of sensitivity through spectral averaging. Furthermore, the units do not utilize any hygroscopic optical components or internal lasers and beamsplitters.
Principle of operation
EP-NIR spectroscopy relies on a simple yet flexible and efficient photometric design whereby the incoming near-infrared beam from a sample is imaged onto a diffraction grating. The dispersed light from the grating is then imaged onto the surface of an encoder disk, which is spinning at 6000 rpm (100 Hz) providing fast, real-time detection. The encoder disk has a series of reflective tracks, which are spatially located within the dispersed grating image to correspond to the wavelengths and wavelength regions used for the analysis. Each track has a gray-scale pattern that produces sinusoidal modulation for each individual wavelength. The reflected beams are reflected onto a single point detector, which generates a discrete interferogram, thereby allowing the treatment of a discrete Fourier transform to obtain quantitative results.
Experimental
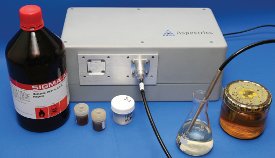
Figure 1 - MC2750 with DIP probe.
An EP-NIR analyzer (Aspectrics, Middleton, WI) covering the 1375–2750 nm spectral range for access to combination bands information was coupled to a highly stable, external halogen NIR source and an extended range 2-mm pathlength process transmission multimode fiber probe in order to develop a calibration for the measurement of the % volume of biodiesel in various diesel blends (Figure 1). Samples of B0, B10, B20, B90, B95, and B100 diesel blends were gathered and analyzed in several replicates. Focus was on the most common blending ranges, B0–B20 and B90–B100. The resulting spectra were randomly split into two groups: the first one was used to develop a calibration model and the other to validate the model to determine the standard error of prediction. Both principal components regression (PCR) and partial least squares (PLS) chemometrics methods were used to develop calibration equations.
Results and discussion
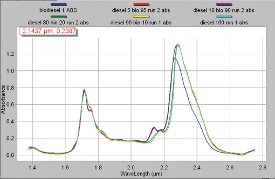
Figure 2 - MC2750 spectra of B0, B10, B20, B90, B95, and B100.
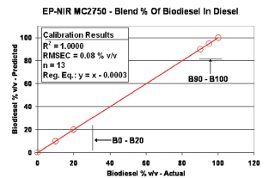
Figure 3 - Measurement of biodiesel % vol. in blend—calibration results.
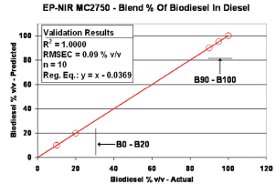
Figure 4 - Measurement of biodiesel % vol. in blend—validation results.
The measurement of biodiesel percentage volume in the final blend can easily be done using chemical information characteristic of and specific to the biodiesel product (Figure 2). During the analysis, the PLS model performed marginally better than the PCR model, allowing for a validated standard error of prediction for the measurement of biodiesel in a blend of root mean square error of prediction (RMSEP) equaling 0.09% volume (Figures 3 and 4). This guarantees, at a 99.9% confidence level, measurement of biodiesel percentage volume in blends of finished product with an accuracy of ±0.27 percentage volume. The EP-NIR analyzer was also able to identify the key chemical substances separating biodiesel fuels and diesel fuels, namely the ester carbonyl organic functional group.
Summary
Determining the percentage volume of biodiesel in diesel fuel blends is particularly important in order to foresee and minimize any potential consequences in engine performance and emissions. Diesel blend detection is also necessary to specify the purity level of the blend and address any possible quality issues.
An experiment was performed to test the capability of EP-NIR spectroscopy to measure the percentage volume of biodiesel in B10–B100 diesel blends. The results from this experiment show that EP-NIR spectroscopy, together with an industrial NIR source and an extended range 2-mm pathlength process transmission multimode fiber probe, is an effective alternative to FT-NIR technology, which is capable of accurately and effectively measuring the biodiesel percentage volume in final diesel blends.
Mr. Beauchaine is International Sales Engineer, Aspectrics, 3510 Parmenter St., Middleton, WI 53562-1535, U.S.A.; tel.: 608-831-2507; fax: 847-235-3130; e-mail: [email protected].