Scientists at the Institute of Ion Physics at the University of Innsbruck (Austria) started their research on proton transfer reaction-mass spectrometry (PTR-MS) in the early 1980s, including hollow cathode discharge and ion mobility drift tube studies. In 1998, the technology had evolved, and IONICON Analytik (Innsbruck, Austria) was able to sell the first commercial PTR-MS instrument. Today, about 200 PTR-MS instruments are in operation worldwide.
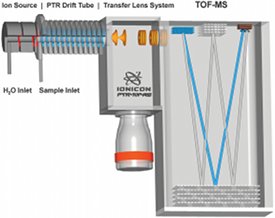
Figure 1 - Schematic illustration of a PTR-TOF 8000 (IONICON Analytik) with hollow cathode ion source, drift tube, transfer lens system, and mass analyzer (left to right).
The PTR-MS principle has been explained in detail in numerous publications.1 In short, water vapor is converted in a specially designed hollow cathode (HC) ion source to H3O+. With this process, purity levels of over 99% can be reached; no mass filter is needed in order to select these primary ions for further reactions. Similar technologies, such as selected ion flow tube (SIFT)-MS, require a mass filter, which results in the heavy loss of primary ion intensity. Attached to this HC ion source is a drift tube that is continuously flushed with the gas to be analyzed. The proton affinity (PA) of water is 691 kJ/mol,2 i.e., it lies well above the PA of all common air constituents (N2, O2, Ar, CO2, etc.). Since proton transfer from H3O+ can only take place with molecules possessing a higher PA than water, the air itself acts as a buffer gas, and no additional gas supply (e.g., He for SIFT instruments) is needed. On the other hand, most volatile organic compounds (VOCs) have a higher PA than water; thus proton transfer takes place with nearly unit probability upon collision with H3O+. Subsequently, the protonated sample molecules are analyzed in a common mass spectrometer (see Figure 1).
Proton transfer is a very soft chemical ionization method, i.e., fragmentation of product ions upon proton transfer is largely suppressed. This leads to very clear mass spectra and enables the direct determination of absolute concentrations of compounds in the sample gas from the measured count rate in the mass spectrum.
In summary, PTR-MS leads to extremely high sensitivity (as a result of the high primary ion intensity and unit reaction probability), real-time quantification (because of the well-known conditions in the drift tube), and response times in the 100-msec region (due to the continuous sampling).
Recent technical developments in PTR-MS
One recent development in PTR-MS was the introduction of time-of-flight (TOF) mass analyzers.3 Although quadrupole mass filters offer the advantages of low weight, low cost, and robustness, time-of-flight mass analyzers provide extremely high mass resolution and the ability to acquire whole mass spectra in seconds. The PTR-TOF 8000 achieves a mass resolution up to m/Δm = 8000, allowing most isobaric VOCs to be distinguished easily. In the study reported in Ref. 3, protonated ketene at 43.0184 m/z and the isobaric compound propene at 43.0548 m/z were observed as two clearly separated peaks, whereas a quadrupole-based instrument would be able to measure only one concentration value on nominal mass 43 m/z.
Following positive feedback from users on the PTR-TOF 8000, a TOF-based PTR-MS model with a reduced mass resolution of about 2000 m/Δm was developed, with increased sensitivity by a factor of five.4 The instrument is well-suited for applications in which high-end mass resolution is not necessarily needed, but a detection limit in the single-digit parts-per-trillion volume (pptv) level is crucial (e.g., detection of trace substances in the high mass range where the probability of multiple isobaric molecules is usually low).
A major development in primary ion preparation is the use of switchable reagent ions (SRI), i.e., the primary ions can be switched from H3O+ to O2+ and NO+ within seconds.5 Therefore, with O2+ a series of molecules can be ionized that have a lower PA than water and would not be seen by proton transfer ionization. The important compounds ethylene and acetylene, for example, cannot be ionized via proton transfer from H3O+. However, their ionization energies are 10.5 and 11.4 eV, respectively, which means that charge transfer takes place when O2+, with its 12.06-eV ionization energy, is utilized as the primary ion. On the other hand, NO+, which is produced from air (i.e., no external gas supply is needed), reacts differently with many isomeric compounds, e.g. ketones and aldehydes. According to Ref. 6, NO+ interactions with aldehydes follow the reaction: NO+ + XH → X+ + NOH, whereas ketones follow the reaction: NO+ + XH → XH+ + NO (and clustering). This means that with PTR+SRI-MS, isomeric compounds on different nominal masses can be detected and identified unambiguously, even when a simple quadrupole mass filter is used.
In the framework of this SRI development, the PTR source itself was also improved. Measurements on trichlorobenzene5 demonstrate that the linearity between the actual concentration and the measured count rate on the detector is over seven orders of magnitude (in concentration) and down to a detection limit of 890 ppqv (parts-per-quadrillion).
All of the above-mentioned developments significantly improve PTR-MS instrument performance. The instruments require gas phase samples; liquids can only be analyzed indirectly via their headspaces. The direct aqueous injection (DAI) device overcomes this limitation—It is possible to directly analyze liquids with a response time of about 20 sec and a detection limit of about 100 parts per trillion weight (pptw).7
Applications
The majority of scientists who use PTR-MS instruments for their research are atmospheric chemists. The extremely high sensitivity and the fact that no sample preparation is necessary make PTR-MS an effective tool for the quantification of environmentally relevant trace substances. The system is able to detect and monitor pollution compounds down to pptv levels, even at elevated heights.
Food and flavor scientists benefit from the technique’s real-time quantification with very short response times, which allows for nosespace analysis during eating or drinking, and the measurement of low-concentration flavor compounds directly in the headspace of a product. Additional fields of application are biology, biotechnology, process monitoring, and medical research (breath analysis).
Although several military institut ions own and deploy PTR-MS instruments for the detection and analysis of illicit substances such as chemical warfare agents, the field is largely unexplored. Extensive testing has been done on explosives (in the gas4 and in the liquid8 phase via DAI), chemical warfare agents (CWAs),9 and drugs.10
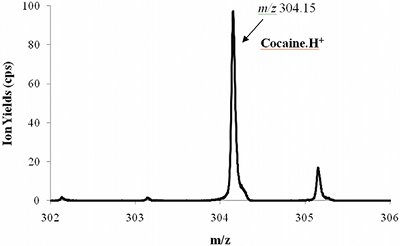
Figure 2 - Part of a PTR-TOF 8000 mass spectrum while analyzing the headspace of about 50-mg cocaine powder.
Figure 2 shows a measurement performed on the headspace of cocaine at room temperature. It can be seen that, even without any preparation or preconcentration of the sample, the signal intensity is more than sufficient for detection. Furthermore, the high mass resolution of the TOF instrument makes substance identification very accurate and reduces false alarms when the instrument is used for detection in real-life scenarios. Similar results have been obtained for other drugs, such as heroin, morphine, and codeine.
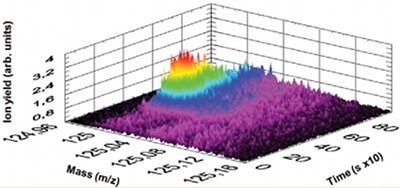
Figure 3 - Measurement of the concentration of a CWA analogue spreading in laboratory air as a function of time.
One application in which very short response times are needed is the monitoring of heating, ventilation, and air conditioning (HVAC) systems. In the case of a CWA attack, the alarm has to be set at a very low concentration in real-time while suppressing false alarms. Figure 3 depicts a measurement of laboratory air. After about 200 sec, a vial containing a CWA analogue is opened. The PTR-MS instrument immediately detects an increasing count rate on the exact mass of the substance. After about 400 sec, the vial is closed, and the HVAC system of the laboratory slowly decreases the CWA concentration in the room, all of which can be observed in real time.