Gas chromatography has become one of the most common analytical techniques in the laboratory today. Its versatility in analyzing volatile and semivolatile compounds gives it particular prominence in the environmental, forensic science, food safety, and petrochemical industries, where samples are often complex and contain a variety of organic constituents that can complicate separation and detection. Sample preparation and methods of identifying eluents have progressed alongside developments of the column and stationary phase materials.
Column development
The concept of GC was first discussed by A.J.P. Martin et al. in 1941, and the possibility of replacing the liquid phase in liquid/liquid partition chromatography with a gas was first suggested.1 Since then, there has been considerable development of the technique, with two main column types, packed and capillary, being available with most stationary phases.
Packed GC columns consist of a 5–10 m stainless steel or borosilicate glass column filled with an inert material, typically silica, which acts as a scaffold for the stationary phase. Packed columns can be made to any practical length, although longer columns require higher pressures, making borosilicate glass unsuitable for the casing material. Packed columns are still commercially available, but their use has been largely replaced.2
Capillary columns are made from stainless steel, quartz, or silica, and possess a narrow internal diameter upon which the stationary phase is applied as a thin film. The length of a capillary GC column can vary hugely, tending to be between 10 and 100 m, with more complex separations supporting longer columns. Separations performed on a capillary column, using an optimized protocol, produce high-quality narrow peaks that can allow sensitive isolation and detection of closely related eluents.2
Capillary columns—technical considerations
The separation power of a capillary column depends on several factors, with the ultimate goal being to achieve the efficient exchange of analyte molecules between stationary and mobile phases. The kinetic energy of a system at higher temperatures favors a rapid exchange of particles between the phases, with typical GC protocols calling for temperatures between 250 and 350 °C. Peaks produced at these temperatures are ideal for downstream, on-line detection methods, such as flame ionization detection (FID) and mass spectrometry (MS).
Gas chromatography protocols place columns under significant strain, with the ramped heating profiles changing column temperatures from 40 up to 350 °C in a few minutes and maintaining these high temperatures for the duration of the run, typically 10–60 min (40–60 min duration of the separation). These columns are then often rapidly cooled to reduce the time between samples and increase productivity. Such heating profiles cause an eventual breakdown of the column coating materials, typically polyimide, leading to brittle columns and causing column bleed.
These phenomena are especially characteristic in high-throughput chromatography applications, where a great many samples are run in an automated or high-sample-volume capacity. It is not uncommon for a column to be replaced due to loss of the polyimide coating, resulting in a brittle or broken column before the phase has deteriorated sufficiently to necessitate replacement. Consequently, column efficiency can be decreased, producing poor peaks and thus poor separations.
Taken to the extreme, this column breakdown can lead to contamination or loss of samples, requiring complete reprocessing. Stationary phase materials are often stable for considerable periods; however, exposure to high-temperature applications and ramped temperature changes can cause column bleed, where stationary phase contents contaminate the eluent peaks and reduce sensitivity due to high baselines and low signal-to-noise ratios.
This article discusses the development and benefits of columns such as Thermo Scientific TraceGOLD metal columns (Thermo Fisher Scientific, Atlanta, GA), which are resistant to high fluctuating temperatures and are able to operate easily at higher sample throughput rates.
Metal columns
Metal-clad GC columns are designed and tested for their resistance to the rapidly changing temperatures of a GC protocol, and to withstand the sustained high temperatures over the duration of a sample run. In addition, a metal GC column provides superior resistance to highly active samples and analytes, reducing the on-column reactions that can compromise a separation. This added flexibility and stability gives the analyst the ability to operate high-throughput protocols, such as fatty acid methyl ester (FAME); simulated distillation (simdist); and polyaromatic hydrocarbon (PAH) analyses, at high temperatures.
- FAME analysis using a metal column. Fatty acid methyl esters are produced as reaction intermediates, with their precursor fatty acids produced as a result of the hydrolysis of triacyl glycerides into glycerol and three fatty acid chains. These fatty acid molecules are subsequently reacted with alcohol molecules, either as part of a planned manufacturing process or as a natural oxidative process within a foodstuff. Sensitive identification and quantitation of these molecules are important in the production of biodiesel and in food safety quality control analyses. Such sample analyses are commonly performed as part of an on-line production process or by external contractors and, as such, are high-throughput techniques demanding rapid turnover.
An experimental mixture of FAMEs, ranging from C8 to C28, was analyzed on a metal column with a wax stationary phase. Eluted compounds were detected by an FID analyzer. The full experimental conditions are given in Table 1.
Table 1 - Experimental conditions for FAME analysis using a TraceGOLD GC column
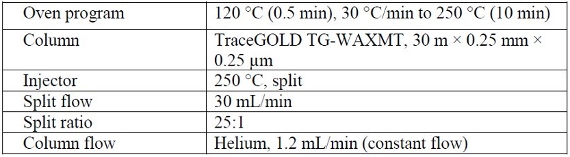
Analysis of this mixture of FAMEs yielded results that were comparable to existing capillary columns, demonstrating that use of a metal column produced similarly high-quality data, while being more robust and resistant to repeated column use (Figure 1). The significant extension of column lifetime, obtained through use of a metal-clad column, provides greater return on investment for high-throughput analytical laboratories.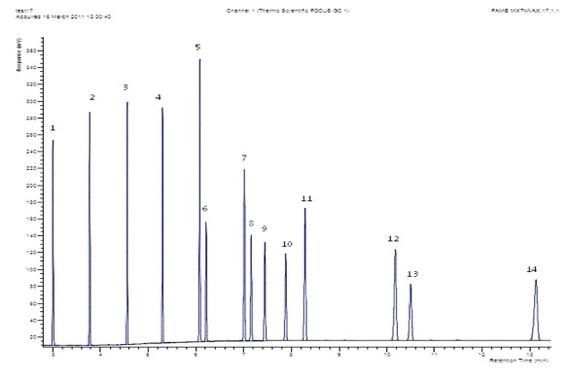
Figure 1 - Chromatogram of 1% C8–C24 FAME solution separated on a TG-WAXMT column with list of FAME identifications. Components: 1) methyl octanoate, 2) methyl decanoate, 3) methyl laurate, 4) methyl myristate, 5) methyl palmitate, 6) methyl palmitoleate, 7) methyl stearate, 8) methyl oleate (cis-9-oleic methyl ester), 9) methyl linoleate, 10) methyl linolenate, 11) methyl arachidate, 12) methyl behenate, 13) methyl erucate (methyl cis-13-docosenoate), 14) methyl lignocerate (methyl tetracosanoate).
- Simulated distillation using a metal column. Simdist is a common technique for assessing the hydrocarbon content of petroleum or crude oil fractions. Using the high temperatures of a GC technique, the hydrocarbons are volatilized and elute from the column according to their boiling point. As such, the individual components of the fraction are released from the column in order of chain length, with longer-chain hydrocarbons having a greater retention time.
The upper temperature limits of silica GC columns are around 350 °C, restricting the identification of hydrocarbon lengths to around C70. Use of a metal-clad column allows oven temperatures to be extended in excess of 400 °C. In this experiment, the analysis temperature was increased to 410 °C, as per the experimental conditions (Table 2).Table 2 - Experimental conditions for simdist using a TraceGOLD GC column
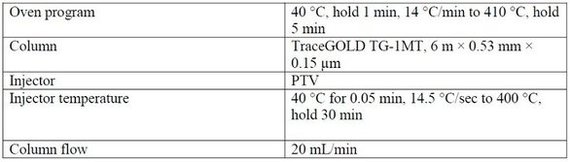
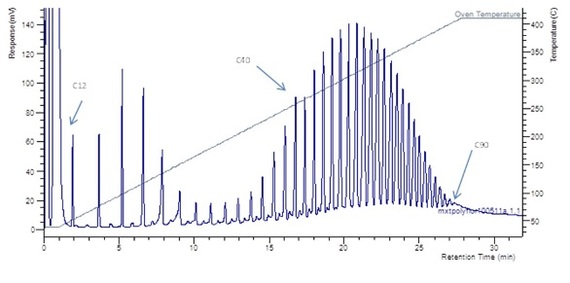
Figure 2 - Simulated distillation of hydrocarbons C12–C90 using a TraceGOLD TG-MT1 metal column.
The data highlighted in Figure 2 demonstrate that a metal-clad GC column can extend the use of applications, such as simdist, to separate and detect a greater range of analytes, in this case extending the detectable range of hydrocarbons from C70 to C90. Further analysis of column bleed levels (data not shown) indicated that the metal columns give lower levels of bleed even at moderate temperatures.
- Analysis of polyaromatic hydrocarbon content using a metal column. PAHs are common environmental contaminants that can cause deleterious toxicological effects as they accumulate in the food chain. It is therefore important that analysis of samples for contamination by these molecules is sensitive and specific. As with analysis for FAME content and simulated distillation applications, samples containing PAH are frequently analyzed in high-throughput protocols, and can suffer from column breakdown as a result.
An experimental mixture of common PAH molecules was separated using a metal-clad GC column, following the experimental conditions outlined in Table 3. Separation of the 16 PAH molecules was achieved using the metal-clad GC column, with results at least comparable with silica column alternatives (Figure 3). Fifteen peaks are seen on the chromatogram as indeno(1,2,3-cd)pyrene and dibenzo(a,h)anthracene elute together, but may be easily separated using MS detection.
Table 3 - Experimental conditions for analysis of PAH using a TraceGOLD GC column
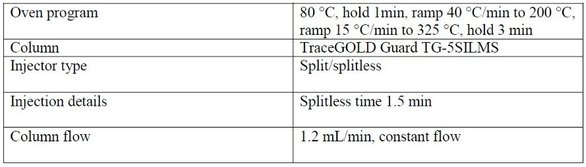
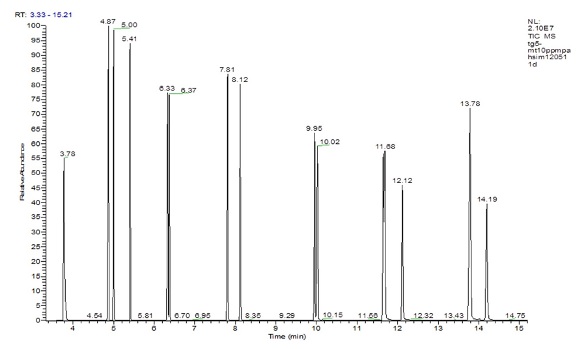
Figure 3 - Sample total ion chromatogram of 16 PAHs analyzed on a TraceGOLD TG-5MT column: 1) naphthalene, 2) acenaphthylene, 3) acenaphthene, 4) fluorine, 5) phenanthrene, 6) anthracene, 7) fluoranthene, 8) pyrene, 9) benzo(a)anthracene, 10) chrysene, 11) benzo(b)fluoranthene, 12) benzo(k)fluoranthene, 13) benzo(a)pyrene, 14) indeno(1,2,3-cd)pyrene, 15) dibenzo(a,h)anthracene, 16) benzo(ghi)perylene.
Conclusion
Gas chromatography is a robust and adaptable technique that is suitable for use with a wide range of volatile and semivolatile organic compounds. It is important that column development and advancements in the technique maintain pace with the rapidly changing environment of the analytical laboratory. The current economic push toward higher-throughput techniques with increasingly complex and active samples can test the integrity of the commonly available capillary columns.
The demands placed upon these columns can cause breakdown of the column material, leading to inefficient separation and/or contamination of the eluent. Metal-clad GC columns, such as Thermo Scientific TraceGOLD, provide the analyst with a more robust and stable column choice. A metal column has the ability to perform high-throughput and high-temperature applications, with reduced risk of column breakdown or column bleed. In addition, column contaminants can be easily removed from metal columns with a high-temperature bakeout, reducing the incidence of sample carryover. A metal GC column is able to perform existing GC applications, without reduction in sensitivity or specificity, but with a significant increase in flexibility and stability of use.
References
-
Martin, A.J.P.; Synge, R.L.M. Biochem. J. 1941, 35, 1358–68.
- Scott, R.P.W. Chromatography Book Series—Gas Chromatography; Library 4 Science Publishing, 2003; www.chromatography-online.org/.
The authors are with Thermo Fisher Scientific, Tudor Rd., Manor Park, Runcorn, Cheshire WA7 1TA, U.K.; tel.: +44 (0) 1928 534110; e-mail: [email protected].