Nuclear magnetic resonance (NMR) spectroscopy is a
powerful analytical technique
that is commonly used to provide
structural information as
well as other chemical metrics
such as sample composition
and highly involved
reaction kinetics. While
NMR is a very informative
tool, its sensitivity is orders of
magnitude lower than other
analytical detection techniques
(e.g., mass spectrometry or UV detection). A number
of approaches have been
developed to improve the
signal-to-noise ratio of an
NMR measurement (i.e.,
high-strength magnets and
cryogenically cooled probes).
These approaches do improve
the S/N, but they also lead to
a significant increase in
instrument cost. An alternative
way to enhance the sensitivity
is to increase the sample
size, but this is not an
acceptable approach for many
studies due to the difficulty of
obtaining the sample (especially
for molecules of biological interest).
In recent years, the use of microcoil
technology has dramatically
improved the sensitivity of NMR
measurements at a considerably lower
cost than cryogenic probes.1
Delivery of the sample to the microcoil
probe is an important issue that
is closely related to the improvement
in sensitivity, since both factors must
be optimized to maximize the
throughput of the system and its utility
to the analyst. In many facilities, a
high-throughput autosampler is
employed, while chromatographic or electrophoretic methods of separation
are used in other facilities. A
seamless analytical method is desired
to optimize the throughput of the
analytical laboratory.
This paper describes the use of microcoil
technology to improve the sensitivity of
NMR measurements. It discusses a
number of sample handling approaches
ranging from microflow injection to
solid-phase extraction (SPE), HPLC,
and electrophoretic migration.
Use of a microcoil to optimize
the sensitivity of an NMR spectrometer for analysis
of microgram and submicrogram
mass levels
In general, the radio frequency (RF)
receiver coil should closely conform to
the sample to ensure good detection
sensitivity. A properly designed NMR probe will maximize both the observe
factor, which is the ratio of the
sample volume being observed
by the RF coil to the total sample
volume required for analysis,
and the fill factor, the ratio
of the sample volume being
observed by the RF coil to the
coil volume. Since the sensitivity
of the coil is roughly
inversely proportional to the
coil size, a probe that employs
a 1-mm RF coil has a mass sensitivity
that is roughly tenfold
greater than a conventional
tube probe that utilizes RF
coils closer to 10 mm in size.
Due to their small size and
patented approach to magnetic
susceptibility matching,
CapNMR™ microcoil probes
(Protasis, Inc., Marlboro,
MA) are easy to use and shim
and typically require only a
handful of the available system
shims to meet resolution specifications.
A detailed discussion
of microcoil NMR design is
presented in Ref. 1.
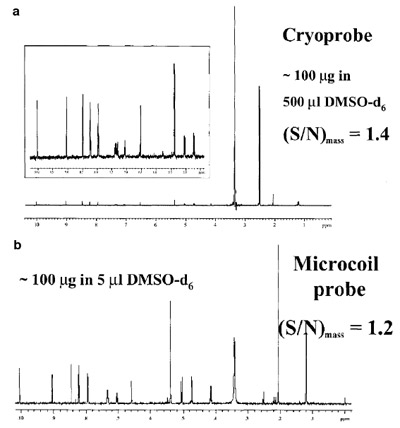
Figure 1 - NMR analysis by cryoprobe and microcoil probe. a) Single
scan using a cryoprobe. The S/N was normalized over the sample
quantity in the active volume (230 μL, Vmrm = 1.5 μL). b) Scan using a
microcoil probe. Reproduced with permission from Dr. Feng Xu, Bristol-Myers Squibb Research Institute (Wallingford, CT).
An example of the utility of
a microprobe is shown in
Figure 1, in which the spectrum of an
unknown is presented using a CapNMR probe and a cryoprobe. While
the S/N for the two spectra are similar,
it is important to note that the
spectrum obtained from the CapNMR probe had considerably less
background signal effects due to the
100-fold reduction in solvent volume
for the mass detected.
Use of a microcoil to obtain
NMR data from biomolecules
The use of a microcoil may be advantageous
for the collection of NMR
spectra of biomolecules since obtaining
a sufficiently large quantity of a
protein (or other large molecule)
may be exceedingly difficult or tedious. In a recent study, Peti et al.2
used the TXI HCN z-grad CapNMR
probe to determine protein folding,
as well as for complete sequence-specific
backbone assignment of a 10-kD protein (TM0979 from Thermotoga
maritima). The probe is well
suited for obtaining the NMR spectrum
of protein samples produced in
nonoptimized, highly automated protein
expression pipelines (where the
amounts of protein produced routinely
have not been sufficient for
traditional 5-mm tube NMR investigations).
The probe opens up a new
avenue for protein folding screening
in the context of structural proteomic
projects.
Peti and coworkers have demonstrated
sequence-specific backbone
assignment using heteronuclear
triple resonance HNCA and
HNCOCA experiments on the CapNMR probe. The group has also
demonstrated TOCSY transfer
between the aliphatic region and
the aromatic region for the first time
in an HCCH–TOCSY experiment
for purposes of side-chain assignments.
This measurement was possible
because of the low power
requirement of the CapNMR probe;
it is a direct result of the mass sensitivity
of the probe and is precluded
in many larger probes due to RF
power restrictions.
High throughput is an
increasingly critical consideration
in many laboratories
in which a large
number of samples are
delivered to the NMR
probe via an autosampler.
The overall time per sample
is a critical issue and is
determined by the residence
time of the sample
in the probe and the sample
preparation and delivery
steps. When the
microcoil probe is
employed, the sensitivity
of the measurement is
increased; thus, the residence
time for data acquisition
can be reduced. Additional
time can be shaved off the time per
sample by optimizing each of the
additional steps in the sample preparation
and transfer process.
One of the most widely used NMR
automation systems employed in
NMR flow injection systems is the model 215 liquid handler (Gilson, Middleton, WI). Protasis and MRM
(Savoy, IL) have developed a means
of loading samples into the CapNMR probe using this liquid handler
in conjunction with a high pressure, HPLC-grade pump. This
has convinced a number of major
pharmaceutical companies to adapt
their flow NMR system to the CapNMR probe.
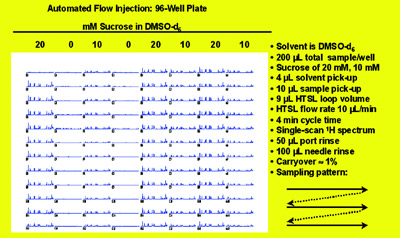
Figure 2 - Automated flow injection for capillary NMR. Reproduced with permission
from Dr. Timothy Peck, Protasis/MRM Corp.
Scientists at GlaxoSmithKline
(Research Triangle Park, NC) have
advanced this development by implementing
a filter maintenance protocol
that provides for significantly increased
mean time to failure. They have
reported error-free injection of thousands
of samples without filter change.
The Inova 600-MHz NMR spectrometer based system (Varian, Palo Alto, CA)
that was used in this study included a
specialized needle (Gilson), custom
loading port (Protasis/MRM), filter,
and CapNMR probe. Figure 2 shows a
collection of spectra from a 96-well
plate. This capillary flow automation
system requires considerably less sample
and solvent and provides less system
downtime and smaller solvent residual
peaks.
The Open Access Automation NMR
system (Protasis/MRM) comprises a
user interface to control the CTC
autosampler (Leap Technologies,
Carrboro, NC) with the NMR. It can
be used with all commercial spectrometers and provides an operator-centric
view of the system for sample control,
report management, and accounting
information. The liquid handler is
specifically designed for microliter volume
samples.