Scientists are increasingly interested
in viewing both ends of the research
spectrum—from cellular and subcellular level observations to tissue,
organ, and whole animal studies. New
hybrid instruments are a meaningful
step toward a deeper understanding of
how macro- and microlevel life
events are intertwined.
Scientists who work with specimens as
varied as Zebrafish, mouse, mammalian
embryo, Arabidopsis, rat brain, C. elegans,
and Drosophila have a common challenge.
These researchers must capture vivid fluorescence images of entire tissues, organs,
systems, or organisms at low magnification.
They also need to capture higher-magnification
images of the cellular
events that are behind the macrolevel
changes. Technology has not made this
easy for developmental biologists, cancer
researchers, cell biologists, genetics
researchers, molecular biologists, ophthalmologists,
neuroscientists, botanists, and
others who perform this kind of imaging.
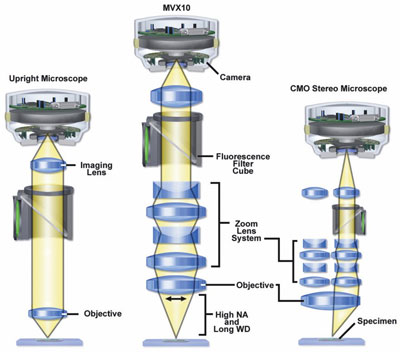
Figure 1 - Optical paths of compound (left), macrozoom
(center), and stereo (right) microscopes. (Figures 1 and 2 courtesy
of Michael W. Davidson, The Florida State University,
Tallahassee, FL.)
Historically, stereomicroscopes
have been used to
view specimens that are too
large or thick for compound microscopes. Stereoscopic
instruments have many useful
features for researchers
studying larger samples in
Petri dishes or as whole animal
preparations. In vivo
processes can in some cases
be followed in real time in
living specimens without
sacrificing the animals. As
the name indicates, stereo instruments offer three-dimensional
viewing, a
benefit for those performing
dissections or animal
surgery. The stereo view is
produced by observing the
same visual field from two
slightly divergent positions,
much as our eyes view
nearby objects, resulting in
the brain’s ability to distinguish depth
(Figure 1). Most stereomicroscopes
also conveniently
allow researchers to adjust
magnification, giving them
the ability to zoom in and
out on the specimen without
extensive refocusing or
repositioning. However,
most stereomicroscopes
have severe limitations for
the researcher using fluorescence.
Stereo optics have a
relatively low capacity to
gather light, as measured by
the numerical aperture
(NA) of their lenses. Low
NA limits the amount of
signal that can be captured
from the sample, making it
extremely difficult to view
or capture fluorescence images with a high signal-to-noise ratio, the chief indicator of a
good fluorescence image.
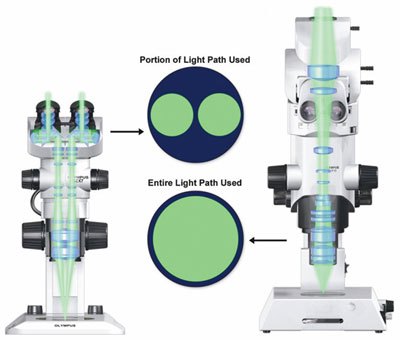
Figure 2 - The common main objective of a stereomicroscope
(left) makes it impossible for either of the instrument’s two
light paths to receive even half of the emitted light coming from
the specimen. The objective (right) of the MVX10 MacroView
zoom fluorescence research microscope (Olympus Corp.,
Tokyo, Japan) is shown for comparison.
The light gathering limitation is critical
and can be further understood by looking
at the two principal designs of the
stereomicroscope. The simpler one is the
Greenough design, in which two narrow,
conventional microscope optical paths are
angled within a single zoom body to produce
a stereo view. Higher-performance
stereomicroscopes typically are of a common
main objective (CMO) or Galilean
design. Light from the sample passes
through the CMO and is imaged by two
parallel zooming “telescopes.” Because the
two light paths view opposite sides of the
CMO, each receives less than half the light
captured from the specimen (Figure 2).
Since a camera can only be attached to
one light path, a great deal of signal from
the specimen cannot be captured for imaging
purposes. Thus, stereomicroscopes, limited in both NA and by separate light
paths, are not optimized instruments for fluorescence microscopy.
Because of the relatively low light gathering
ability of stereomicroscopes, many
biologists have chosen traditional compound
microscopes for fluorescence imaging. However, the design parameters
that make compound microscopes flexible
and functional for high-magnification
imaging tend to also limit their performance
for low-magnification, large-field-of-view imaging. Limitations in NA and
working distance can frustrate low-magnification
fluorescence imaging,
manipulation, and dissection on compound
instruments.
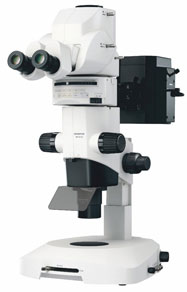
Figure 3 - The MVX10 MacroView
microscope combines the best features of both
stereo and compound microscopes for bright,
crisp fluorescence images from 4× to 250×.
In the end, a number of researchers find
themselves switching between stereo and
compound microscope systems to meet
the requirement of associating cellular
and molecular phenomena with specific
events at the organism level. But combining
the best of both stereo and compound
microscopes would theoretically
be the optimum way to address the need
for low- to high-magnification imaging of live, fluorescing specimens. The optimal
system for fluorescence observation and
recording in intact organisms must combine
maximum detection sensitivity at
the lowest magnifications with a high-magnification zoom for the resolution of
fine details within organs, tissues, and
cells. The MVX10 MacroView zoom fluorescence
research microscope addresses
the tradeoffs between stereo and compound
imaging systems, and was designed
specifically for macro- to microfluorescence
imaging (Figure 3). It offers the
same working distances and large fields of
view as a stereomicroscope, but with a
single, full-objective optical path for
imaging devices, and much higher NAs
for improved resolution and light gathering
ability.
Fluorescence observations ranging from
low-magnification viewing of whole
organisms like Zebrafish to high-magnification
imaging of gene expression
at the cellular level are possible (Figure 4).
With features such as a two-objective
nosepiece, 2× objective lens, and 2× magnification
changing lens, the microscope
can zoom from 4× to 250× (a zoom factor
of 31 times) with high image quality,
regardless of the specimen medium.
Moreover, the 2× objective has a correction
collar that adjusts for up to 5 mm of
aqueous medium above the specimen.
The instrument also has working distances
(WD) that are much greater than
compound microscopes (for instance, 65
mm WD for the 1×/0.25-NA objective)
and its field of view ranges up to 55 mm,
making it easy to observe, track, or work
on large or fast-moving specimens.
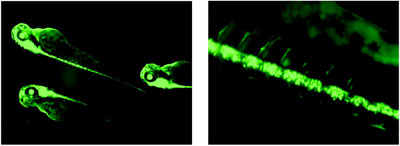
Figure 4 - Images (including zoom image) of transgenic green fluorescence protein (GFP)
Zebrafish with expression in the brain and spinal cord, captured using the MVX10. (Courtesy of
Richard Dorsky, Dept. of Neurobiology and Anatomy, University of Utah, Salt Lake City, UT.)
Its three parfocal objectives—0.63×/0.15
NA, 1×/0.25 NA, and 2×/0.50 NA—are
plan apochromatic to produce the
highest-level image quality with better-quality
chromatic aberration correction.
They offer good transmission and high
signal-to-noise ratios, so that living samples
can be exposed to intense illumination
for much briefer time periods. The
objectives can deliver up to 10 times the
light intensity from a fluorescing specimen
to an image capture device, when compared
with many high-end stereomicroscopes.
The objectives offer high optical
performance through the near-IR range,
making the instrument versatile enough
to address the wide variety of live cell
applications for current and future use.
For visual stereoscopic viewing, the
patent-pending pupil separation
mechanism mimics the natural offset
of the human eyes at a somewhat
lower degree of separation—all by just
moving a slider. The mechanism is
not used when imaging with a camera,
so that 100% of the light output is
always delivered to the image capture
device at the moment of exposure.
Ergonomic features such as tilting eyepieces
make it comfortable for users of
varying heights.
With novel imaging capabilities
becoming available to researchers
through instrumentation, software,
probes, and new methodologies, scientists
will move to the next level in their
understanding of how events on the
cellular and organism levels are related.
Mr. Higgins is Group Marketing Manager,
Olympus America Inc., Scientific Equipment
Group, Two Corporate Center Dr., Melville, NY
11747, U.S.A.; tel.: 631-844-5066; fax: 631-844-5111; e-mail: [email protected].