Ultrasensitive calorimetric instruments for measuring
very small heats of reaction at constant temperature
(isothermal titration calorimetry [ITC]) and
small heat effects induced by temperature (differential scanning calorimetery [DSC]) are becoming
increasingly important in biotechnological studies.
Ultrasensitive calorimetry has gained much interest
among life scientists because it provides information
on the energetics of biopolymer structures, and on
the interactions of biopolymers with other macromolecules
and with small molecules. Since these
recognition and binding reactions are central to all
biological processes, this information is indispensable
for furthering developments in biology, medicine,
and the pharmaceutical sciences.
Today’s ultrasensitive calorimeters provide a unique
approach to analyzing molecular structures such as
proteins, nucleic acids, and their complexes. Our
increased understanding of biomolecular structures
and interactions provide new possibilities for finding
and developing novel biologically active products.
The calorimetric analyses of biomolecules have garnered
much attention due to the fact that structural
information alone is insufficient for understanding
their function. This requires knowledge of the energetics
that determine the structures of biomolecules and
their complexes. The only way to obtain this information
is through direct calorimetric measurements of
the thermal properties of the individual molecular
objects (proteins, nucleic acids, etc.), i.e., their heat
capacities and the heats of their interactions.
Understanding and accurately quantifying such
interactions requires characterizing structural and
stability changes in biological macromolecules, their
mutants, and synthetic analogs. These changes are
induced by various external conditions, or by association
with their binding partners and ligands. While
these processes can be studied by various physical
methods, ultrasensitive calorimetry alone provides
direct information on the energies involved.
The heat effects arising from macromolecular binding
processes are extremely small. Moreover, proteins
and nucleic acids are generally available in
very limited amounts. In order to measure these heat
effects, two types of ultrasensitive microcalorimeters
have been developed. Differential scanning calorimeters
measure the heat effects induced by temperature
variations, and isothermal titration calorimeters
measure the heats of interactions at constant temperature.
These two types of calorimetric instruments
are complementary; only in combination can
information on the energetic bases of the studied
molecular objects and their interactions be obtained.
Researchers understand that these two tools are
complementary: a full thermodynamic description
of the interactions between macromolecules (which
is the basis of all biological functions) can only be
obtained when ITC and DSC are used together to
study biomolecular systems. Using both techniques
to study a biomolecular system in dilute aqueous
solution is important because macromolecular interactions
depend on the exact structure and stability
of the molecules, which in turn depends on the temperature.
Therefore, although ITC provides detailed
information about the strength with which two molecules
bind, DSC studies are required to understand
the thermal properties of the interacting
molecules. Attaining this level of understanding
will particularly impact the pharmaceutical
industry, opening new avenues in the search for
active molecular agents with high binding affinity
and specificity for their target macromolecule.
Ultrasensitive calorimeters dramatically enhance
reliability, baseline reproducibility, and data consistency,
resulting in more precise measurements. In
many instances, these instruments allow scientists
to obtain precise measurements in a single experiment,
making it unnecessary to take a multitude of
measurements, thereby saving time and money.
The Nano DSC III (Calorimetry Sciences Corp.
[CSC], Lindon, UT) enables scientists to maintain
very good baselines. They are able to determine
the heat capacity of the macromolecule of
interest in dilute solution over a broad temperature
range, the changes that occur in the protein
upon binding a specific ligand, and detect which
protein domains are involved in binding a given
ligand. Because these measurements are obtained
over a range of temperatures, they can be used to
make temperature corrections to heat of binding
data obtained using the calorimeter. This correction
allows correlations between the energetics of
binding and the structure of the complex, which
is extremely important in analyzing the action of
various ligands and in searching for biologically
active agents.
While ITC is particularly suitable for following
the energetics of association reactions between
biomolecules, the combination of ITC and DSC
provides a more comprehensive description of
the thermodynamics of the system. Both techniques
allow researchers to use very small samples.
When a system is studied by ITC, the data clearly
tell what is happening, and by knowing the fundamentals
of this, the user can extrapolate to various
conditions. Without the correct DSC data, researchers
cannot make the required corrections to the ITC
data to extrapolate and work with them.
Essentially, ITC is a thermodynamic technique for
monitoring any chemical reaction initiated by the
addition of a binding component. It has become the
method of choice for characterizing biomolecular
interactions. When substances bind, heat is either
generated or absorbed. Measuring this heat permits
the accurate determination of binding constants,
reaction stoichiometry, enthalpy, and entropy,
thereby providing a complete thermodynamic profile
of the molecular interaction in a single experiment.
DSC is used to study thermally induced conformational
changes in biopolymers and lipid membranes,
and ligand binding to biopolymers and biomembranes.
Changes in heat capacity, enthalpy, and
entropy that accompany changes in conformation
or binding are commonly determined. Free energies,
compressibilities, and thermal expansion coefficients
can also be determined by combining DSC with
pressure-volume changes.
The limitations of using ITC alone is that researchers
are making measurements at multiple temperatures and then trying to get heat capacity changes as
a function of temperature from the ITC data. However,
they are not taking into account the change in
the native protein and its heat capacity change as a
function of temperature. Measuring those thermodynamic
parameters accurately requires highly sensitive
DSC with ultrastable baselines.
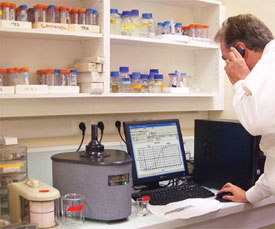
Figure 1 - Nano ITC III ultrasensitive calorimeter.
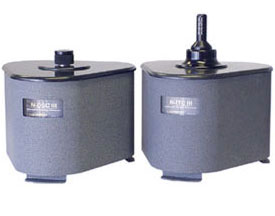
Figure 2 - CSC Nano DSC III and Nano ITC III ultrasensitive
calorimeters.
The CSC Nano DSC III and Nano ITC III are power
compensation calorimeters with cells that are fixed-in-place (see Figures 1 and 2). These high-precision
calorimeters are well suited for biopolymer studies.
Because many biopolymers are expensive or difficult
to obtain, and may behave differently depending on
their concentration in solution, there is a need for
scanning and titration instruments that can make
reliable measurements with very little sample, often
just a few micrograms of biopolymer.
Conclusion
Ultrasensitive ITC and DSC are complementary techniques
that enhance our understanding of structural
and stability changes as biological molecules interact.
Together they form a winning combination that offers
a unique approach to analyzing molecular structures
such as proteins, nucleic acids, and their complexes.
Mr. Sullivan is a writer based in Hermosa Beach, CA, U.S.A.;
tel.: 310-379-0573; e-mail: [email protected].