It is a universal fact substantiated by decades of
biopharmaceutical research: the earlier a disease is
detected, the more likely treatments will be effective
in alleviating or stabilizing the disease process. As a
result, the need for earlier diagnosis to help ensure
positive outcomes has become central to the mindsets
of researchers, clinicians, patients, and third-party
payers.
The pharmaceutical industry is striving to develop
effective new therapies for diseases, ranging from
cancers to cardiovascular and neurodegenerative
disorders to a host of metabolic, infectious, and
genetic conditions, and is placing emphasis on treatments
related to the early detection of disease. The
development of new molecular diagnostic methods
capable of detecting disease at the molecular level
in blood, cerebrospinal fluid, and other body specimens
lies at the core of an emerging revolution in
disease diagnosis. Using specific and targeted protein
and nucleic acid (i.e., DNA and RNA) biomarkers,
clinicians will be able to detect diseases and confirm
diagnoses very early on. Ideally, clinicians may be
able to diagnose even before patients present with
clinical signs and symptoms—when a disease is most
amenable to successful treatment.
Medical schools do a very good job of teaching budding
physicians how to detect diseases when a patient
presents with easily observed classical signs and
symptoms. A host of diagnostic tests, from imaging studies to laboratory-based analyte measurements to
biopsy of affected tissues, are available to supplement
and support clinical findings and to guide physicians
through the decision-making process that leads
to a diagnosis and treatment plan. Yet all of these
diagnostic strategies are often based on discovering
disease that has progressed to the point where it has
already caused irreversible damage to organs, tissues,
and vital biochemical and physiological processes.
Alzheimer’s disease, ovarian cancer, and coronary
artery disease are three potent examples of the need
for a paradigm shift in disease diagnostics. There
is now potential for the detection of protein and
nucleic acid biomarkers with ultrasensitive molecular
diagnostic tools that identify disease-related
changes in biomarker levels, before they manifest
with advanced clinical signs and symptoms secondary
to disease progression.
Alzheimer’s disease often presents with nonspecific
cognitive changes and is very difficult to detect in its
early stages (i.e., mild cognitive impairment, prior to
significant beta-amyloid plaque formation). In fact,
Alzheimer’s disease currently can only be diagnosed
definitively at postmortem examination. Promising
therapies for Alzheimer’s disease now in development
have the potential to be more effective if treatment
can be initiated earlier in the natural history
of the disease. Neurodegenerative diseases such as
Alzheimer’s will likely be more susceptible to drugs
capable of slowing their progression at an early stage,
thereby prolonging the time between diagnosis and the appearance of more debilitating clinical symptoms
that compromise patient function and quality
of life.
Researchers at Northwestern University (Evanston,
IL) have discovered toxic derivatives of beta-amyloid,
one of a family of proteins found in the plaques and
neurofibrillary tangles characteristically found in
the brains of individuals with Alzheimer’s disease on
postmortem evaluation.1 Called amyloid-derived diffusible
ligands (ADDLs), these derivatives are more
neurotoxic than beta-amyloid itself, and could serve
as early indicators of Alzheimer’s disease. Similarly, a
phosphorylated form of the tau protein—also implicated
in the brain pathology linked to Alzheimer’s
disease—called p-tau-231, may be a more sensitive
diagnostic marker than the tau protein itself, since it is
a precursor to tau that appears earlier in the course of
the disease.2,3 Both of these biomarkers are present in
very low concentrations (<1 pmol) in the cerebrospinal
fluid (CSF) of patients with Alzheimer’s disease,
concentrations below the levels reliably detectable
with available assay technology.
The small sizes of ADDLs and p-tau-231 may allow
these molecules to cross the blood–brain barrier,
suggesting that it might be possible to develop an
assay that could detect their presence in blood. This
would allow physicians to rely on a blood sample to
diagnose and monitor the disease, eliminating the
need for the more complicated, potentially risky, and
uncomfortable procedure required to obtain a sample
of cerebrospinal fluid.
Even as the discovery of new biomarkers for cancer
progresses at a promising pace, current assay technologies
lack the limits of detection needed to identify
these biomarkers in biological samples efficiently,
reproducibly, and cost-effectively. Ovarian
cancer is a good example. In nearly two-thirds
of women with ovarian cancer, the
tumor is typically not detected until it has
progressed to an advanced stage, when the
five-year life expectancy is only 12–39%.4,5
Although the five-year survival rate for all
stages of ovarian cancer combined is only
35–38%, if the diagnosis is made early in
the course of the disease, these survival rates
can reach 90–98%.6
Researchers at the Food and Drug Administration
and the National Cancer Institute
have reported that qualitative mass spectroscopy patterns of proteins in
patients’ blood could distinguish between
ovarian cancer and control samples.7
Ongoing research is also focusing on a
protein called inhibin, which antagonizes
the action of another protein, activin, and
may have a role as a potent and specific
biomarker for one form of ovarian cancer.8 Whereas the CA-125 protein, routinely
used to screen and monitor patients
for ovarian cancer, can signal the presence of the most common types of ovarian cancer,
which are epidermal in origin and which represent
about 90% of ovarian cancers, it is not very useful
for detecting the 10% or so of granulosa cell
tumors. In contrast, inhibin levels are increased in
blood samples taken from postmenopausal women
with granulosa cell ovarian tumors. Diagnostic
test results, combining detection of both CA-125
and inhibin, have been presented that may detect
95% of all ovarian cancers with 95% specificity.8
More precisely, it has been reported that inhibin
is almost 100% accurate for granulosa cell ovarian
tumors, CA-125 is about 60% accurate for epidermal
ovarian tumors, and an assay of CA-125 plus
inhibin is about 90% accurate for epidermal ovarian
tumors. Inhibin and other potential protein
biomarkers for ovarian cancer (e.g., soluble epidermal
growth factor receptor [EGFR], Mullerian
inhibitory substance) are present in extremely low
concentrations in blood that cannot be measured
quantitatively with current methods.
Coronary artery disease represents another diagnostic
area in which ultrasensitive protein biomarkers may
help guide clinical decision-making. Approximately
8 million patients with chest discomfort present to
the emergency department annually. Measurements
of blood levels of a very specific protein (i.e., cardiac
troponin) are the gold standard test for diagnosing an
acute myocardial infarction (MI). Currently, the troponin
level rises as heart muscle breaks down, but the
troponin level cannot be detected as abnormal until
the level becomes >0.01 to 0.10 ng/mL, depending
on the given assay. It takes 4–6 hr, from the first cardiac
symptoms, for the troponin level to rise above
this abnormal threshold. The ability to detect an
initial burst of troponin from the myocardial cell,
appearing in the blood within 1 hr after MI symptoms, may enable a more rapid diagnosis and subsequent
treatment of MI. Likewise, a more sensitive
troponin assay may permit clinicians to differentiate
unstable angina (UA) from less dangerous forms of
chest pain. Patients who are experiencing UA are at
high risk of having another cardiac event within the
next 30 days. However, frequently UA goes undetected
because troponin levels remain below the current
thresholds of abnormality. These UA patients
may be sent home without the administration of any
form of therapy, often with fatal consequences (i.e.,
up to 10–20% mortality). An ultrasensitive cardiac
troponin test capable of detecting very low levels of
troponin (e.g., limit of detection [LOD] of 0.0001
ng/mL with good precision [<20% CV] at the LOD)
may be useful in the diagnosis of unstable angina and
to identify patients that require treatment and hospitalization
even though their troponin level never
reaches the current MI threshold.
Potential technology platform solution
The development of the tools and technology
needed to bring molecular diagnostic tests into the
clinical mainstream will have a profound effect on
the discovery of novel biomarkers, which will, in
turn, accelerate drug discovery research and enhance
efforts to screen targeted populations for a variety
of common medical disorders and risk factors of disease.
The availability of a highly sensitive, automated,
quantitative, cost-effective, and easy-to-use
diagnostic platform capable of rapidly and reliably
identifying both protein and nucleic acid biomarkers
(often present in minute concentrations in biological
specimens) could deliver the power of molecular
diagnostics to both reference laboratories and community
hospital laboratories. In addition, for many
disorders, biomarker discovery research, using this
tool or other methods, may identify highly specific
protein and nucleic acid markers that play key roles
in the targeted detection of early-stage pathology.
Also, molecular diagnostic tools that are capable of
performing multiplexed assays—that is, tests that
simultaneously detect multiple protein biomarkers
and/or genetic mutations (i.e., single nucleotide
polymorphisms [SNPs] of DNA and RNA)—will
save time and resources and, more importantly, allow
clinicians to detect patterns of disease-related markers.
Multiplexing will enable diagnoses based on a
more informative assessment of panels of biomarkers
that could signal the presence of or predisposition
to a disease and also provide information on disease
stage and aggressiveness that could contribute to the
determination of prognosis and the course of effective
patient management.
The Verigene® System, a technology platform
based on the laboratory-proven nanoparticle (gold)
probe and Biobarcode™ technology (Nanosphere,
Inc., Northbrook, IL), enables ultrasensitive, multiplexed
detection of both protein (Biobarcode
detection) and nucleic acid (PCR-less, direct
genomic detection) biomarkers, using enhanced
signal amplification techniques (see Figure
1).
The scientific basis of the technology came from
two world-renowned Northwestern University
professors, Chad A. Mirkin, Ph.D., and Robert
L. Letsinger,
Ph.D. Dr. Letsinger is known internationally
for developing the chemistry behind
the modern-day “gene machines.” Dr. Mirkin is a
pioneer in the development of ultrasensitive and
highly selective assays based on nanostructures.
He is currently the Director of the Northwestern
University International Institute for Nanotechnology
and is internationally recognized as one
of the most influential figures in nanotechnology.
Combining their expertise and resources, they developed the foundation for the technology in
the Verigene System, including the processes to
create the Biobarcode (gold) nanoparticles.
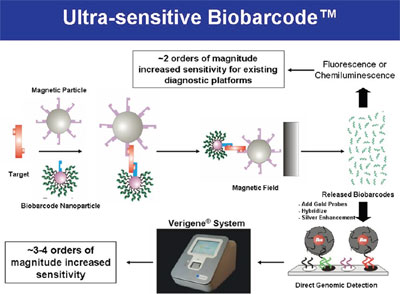
Figure 1 - Schematic of ultrasensitive protein detection with gold nanoparticle
probes and single-stranded oligonucleotide bar codes (Biobarcode detection).
Note that direct genomic detection is a part of the Biobarcode procedure
(see lower right corner of figure). Instead of “released bar codes,” extracted
and purified DNA or RNA (from about 1 mL of blood) can be introduced into
the direct genomic detection hybridization, allowing PCR-like sensitivity for
detection of genetic polymorphisms and mutations (SNPs).
The combination of gold nanoparticle and
Biobarcode
technology permits the detection of very
low levels of proteins—levels far below those detectable
using routine ELISA, Western blot, or other
currently available assay methods. The Biobarcode
nanoparticle assay achieves signal amplification in
two ways: 1) the multiplicity of identical bar codes
(about 100–1000) released as a result of each target
protein molecule that is captured (note: multiplexing
occurs here by changing the capture antibodies
and bar-code sequence for each specific analyte in a
panel), and 2) a silver-enhanced optical detection
method. In comparison with conventional ELISA-based
diagnostic assays, Biobarcode technology is
1000–10,000 fold more sensitive, with a detection
limit in the attomolar range.
Conclusion
The need for ultrasensitive detection of biomarkers
is presented, using only three examples (i.e.,
Alzheimer’s disease, ovarian cancer, and coronary
artery disease). Of course, there are a myriad of other
clinical applications in which enhanced diagnostic
assay sensitivity could improve the health of at-risk
populations worldwide. For example, the following is
an incomplete list of general-need areas:
1. Oncology
- Prostate cancer screening
- Prostate cancer recurrence, after surgery or radiation therapy
- Ovarian cancer
- Other cancers (lung, pancreatic, colon, uterine, renal, bladder)
2. Neurodegenerative diseases
- Alzheimer’s disease9
- Parkinson’s disease
- Other protein folding disorders
3. Cardiovascular diseases
- Myocardial ischemia (coronary artery disease)
- Chronic heart disease (silent ischemia, congestive heart failure)
4. Infectious diseases
- Human immunodeficiency virus (HIV)
- Herpes simplex virus (HSV)
- Respiratory panel
- Transmissible spongioform encephalopathies (TSE)—prion proteins
- Variant Creutzfeldt-Jakob (vCJD) disease—humans
- Bovine spongiform encephalopathy—cows
- Chronic wasting disease—deer and elk
- Scrapie—sheep
- Sepsis
5. Genetic abnormalities
- Down syndrome
- Hypercoagulability (Factor V Leiden, Factor II, MTHFR)
- Cystic fibrosis
- Warfarin metabolism (CYP 2C9, VKORC1).
6. Renal diseases
7. Stroke
8. Blood screening
9. Traumatic brain injury (TBI).
The nanoparticle probe strategy offers several
unique advantages when compared with traditional
ELISAs (for protein detection) and PCR-based
target amplification methods (for genetic detection).
The strategy eliminates the need for other,
more costly and time-consuming approaches, such
as PCR amplification for current genetic detection
and qualitative mass spectroscopy or immuno-PCR for current protein detection.
With direct genomic detection—which is the
first commercial application of the nanoparticle
probe technology—signal amplification
without the need for PCR in an automated,
cost-effective system will provide an economically
feasible, easy-to-perform method of detecting
genomic markers. The first products based
on the direct genomic technology will include
assays for hypercoagulability, cystic fibrosis, and
warfarin metabolism.
Ultrasensitive detection of protein and nucleic
acid biomarkers will not only enable screening for
and early detection of diseases with established
diagnostic biomarkers, but will also improve biomarker
discovery research for both clinical diagnostic
applications and drug development. It will
also play a role in advancing pharmacogenomics
and efforts to improve blood screening. As new
biomarkers are identified and used in the clinical
arena to diagnose, stage, and monitor disease,
the simplicity and efficiency of ultrasensitive
detection technology should make it possible
for smaller, community-based hospitals to access
and implement the molecular tools and strategies
that are at the forefront of advances in molecular
diagnosis, risk stratification of diseases, and targeted
therapeutics.
References
- Lambert, M.P.; Barlow, A.K.; Chromy, B.A.; Edwards, C.; Freed, R.; Liosatos, M.; Morgan, T.E.; Rozovsky, I.; Trommer, B.; Viola, K.L.; Wals, P.; Zhang, C.; Finch, C.E.; Krafft, G.A.; Klein, W.L. Diffusible, nonfibrillar ligands derived from Ab1→42 are potent central nervous system neurotoxins. Proc. Natl. Acad. Sci.1998; 95, 6448–53.
- deLeon, M.J.; Segal, S.; Tarshish, C.Y.; DeSanti, S.; Zinkowski, R.; Mehta, P.D.; Convit, A.; Caraos, C.; Rusinek, H.; Tsui, W.; Saint Louis, L.A.; DeBernardis, J.; Kerkman, D.; Qadri, F.; Gary, A.; Lesbre, P.; Wisniewski, T.; Poirier, J.; Davies, P. Longitudinal cerebrospinal fluid tau load increases in mild cognitive impairment. Neurosci. Lett. 2002, 333, 183–6.
- Hampel, H.; Buerger, K.; Zinkowski, R.; Teipel, S.J.; Goernitz, A.; Andreasen, N.; Sjoegren, M.; DeBernardis, J.; Kerkman, D.; Ishiguro, K.; Ohno, H.; Vanmechelen, E.; Vanderstichele, H.; McCulloch, C.; Moller, H.J.; Davies, P.; Blennow, K. Measurement of phosphorylated tau epitopes in the differential diagnosis of Alzheimer disease. Arch. Gen. Psychiatry2004, 61, 95–102.
- http://ovariancancer.jhmi.edu/prognosis.cfm.
- http://ovariancancer.jhmi.edu/earlydx.cfm.
- http://nlm.nih.gov/medlineplus/ency/article/000889.htm.
- Petricoin, E.F.; Ardekani, A.M.; Hitt, B.A.; Levine, P.J.; Fusaro, V.A.; Steinberg, S.M.; Mills, G.B.; Simone, C.; Fishman, D.A.; Kohn, E.C.; Liotta, L.A. Use of proteomic patterns in serum to identify ovarian cancer. The Lancet2002, 359, 572–7.
- Robertson, D.M.; Pruysers, E.; Burger, H.G.; Jobling, T.; McNeilage, J.; Healy, D. Inhibins and ovarian cancer. Mol. Cell Endocrinol. 2004, 225, 65–71.
- Georganopoulou, D.G.; Chang, L.; Nam, J.-M.; Thaxton, C.S.; Mufson, E.J.; Klein, W.L.; Mirkin, C.A. Nanoparticle-based detection in cerebral spinal fluid of a soluble pathogenic biomarker for Alzheimer’s disease. Proc. Natl. Acad. Sci. 2005, 102, 2273–6.
Dr. Shipp is Vice President of Medical and Regulatory Affairs,
Nanosphere, Inc., 4088 Commercial Ave., Northbrook, IL
60062, U.S.A.; tel.: 847-400-9115; fax: 847-400-9199;
e-mail: [email protected].