Fluorescence microscopy requires an intense light
source at the specific wavelength that will excite fluorescent
dyes and proteins. The traditional method
employs a white light, typically from a mercury or xenon arc lamp. Although such broad-spectrum lamps can generate ample light at desired wavelengths,
only a small percentage of the projected
light is useful in any particular application. The
other wavelengths need to be suppressed to avoid
background noise that reduces image contrast and
obscures the fluorescent light emissions.
This process of suppressing extraneous light is complex,
expensive, and only partially effective: Even
after decades of refinements, the best filters are not
100% successful at blocking the bleed-through of
nonspecific photons. Some mitigation techniques
end up not only suppressing peripheral light, but
also significantly diminishing the intensity of the
desired wavelengths. To address the root cause of the
problem—the presence of nonspecific photons—a
radically different approach is needed.
Recent advances in high-performance light-emitting
diode (LED) technology have enabled
the practical implementation of this theoretical
model. High-intensity monochromatic LEDs are
now available in a variety of colors that match the
excitation bandwidth of many commonly used fluorescent
dyes and proteins.
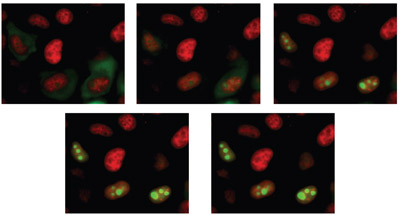
Figure 1 - Time-lapse images of Hela cells labeled with DS Red and expressing mutant YFP.
Carl Zeiss MicroImaging (Thornwood, NY) has
incorporated this LED technology in the Colibri illumination system, a light source system for widefield fluorescence microscopy that uses specific
wavelength windows with much less need
to suppress unwanted peripheral wavelengths
from a white light arc lamp. The modular system
employs up to four LEDs, each individually and
instantly controlled by electrical current without
any of the mechanical switching devices such as
filterwheels or shutters required by traditional
illumination systems. LEDs of different colors can
be used in combination, giving users the option
of seeing multiple fluorochromes simultaneously
or rapidly capturing sequential images of each
fluorochrome (Figure 1).
Fluorescence microscopy utilizes optical filters to
separate excitation light from the emitted fluorescence,
which is observed visually or detected by
a camera equipped with a charge-coupled device (CCD) or other detectors. Fluorescence microscopy
is an increasingly widespread medical and
biological laboratory technique that provides
highly sensitive detection for medical diagnostics
and allows for the detection of cellular components
and inter- and intracellular communication.
Fluorescence microscopy is capable of detecting
single molecules and submicroscopic structures
that are too small to be resolved by other conventional
microscope techniques.
The availability of (green) fluorescent proteins
(GFPs) derived from jellyfish, corals, etc., and its color-shifted genetic derivatives has greatly expanded
the use of fluorescence microscopy during the past
10 years. Fluorescent proteins such as GFP are less
phototoxic than the small fluorescent molecules in
most chemical dyes such as fluorescein isothiocyanate
(FITC), which can harm the specimen when
illuminated during live cell fluorescence microscopy.
This attribute of fluorescent proteins has inspired the
development of highly automated time-lapse live cell imaging systems.
Even more important is the fact that fluorescent
proteins can be expressed by the cells, while other
fluorescent dyes usually cannot penetrate the membranes
of living cells. This limits their use to mainly
fixed cells or the need for microinjection, electroporation,
etc.
The most basic requirement of a fluorescence microscopy light source is closely matching the
excitation wavelength of the fluorochrome to
achieve a high-contrast image, i.e., an image with a
high signal-to-noise ratio. Wavelengths that match
the fluorochrome strengthen the signal, but any
peripheral wavelengths produce background noise
that can overshadow the signal emitted by the
object of interest.
A second and related requirement is illumination
intensity, i.e., the number of photons specific to
the excitation wavelength that reach the specimen.
The human eye is less sensitive than most automated
detection systems, and therefore applications
involving visual observation typically require
higher levels of illumination intensity. On the other
hand, lower intensity is necessary for live cell imaging applications to protect against photobleaching
and phototoxicity.
Intensity of illumination and signal intensity do not
just follow a linear correlation. Saturation effects
and dark states become more and more important
with increasing illumination intensity.
An additional consideration is protection of the
specimen, especially in live cell imaging applications.
The dangers of overexposure to light have
already been mentioned: phototoxicity of the specimen
and photobleaching of the fluorescent dye or
protein. Overexposure can be avoided by attenuating
the light intensity and by limiting the duration
of the illumination to exactly the exposure time of
the sensor. The cells also need to be protected from
the heat generated by light source lamps and from
the vibrations caused by mechanical filtering and
switching devices.
Another factor to consider when evaluating light
sources is the lifetime and stability of the lamp.
Some light sources exhibit short-term intensity
fluctuations and a substantial deterioration of
performance over time. Limited life span results
in more frequent bulb replacements, and poor
stability diminishes the reproducibility of illumination
conditions.
Advantages of LED in
fluorescence microscopy
Now that high-performance LEDs provide sufficient
intensity at the specific wavelengths required for
many applications, fluorescence microscopy is able
to take advantage of the benefits of LEDs, including
their compact size, low power consumption,
minimal heat output, fast switching and adjusting
properties, high emission stability, and extremely
long life span.
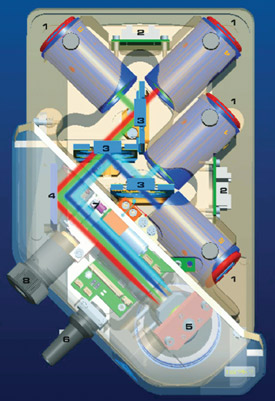
Figure 2 - The Colibri LED light source system employs a
flexible modular design and up to four LED modules that can
be used simultaneously in Colibri.
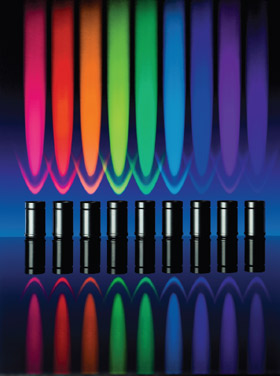
Figure 3 - Ten different LED modules that can be easily
exchanged are currently available for Colibri, from UV to
dark red.
The Colibri LED light source system employs a
flexible modular design and up to four LED modules
that can be used simultaneously in the system
(Figure 2). The beam paths from each module are
steered into the microscope with a series of beam
combiners, and 10 different LED modules are currently
available for Colibri, from UV to dark red
(Table 1 and Figure 3).
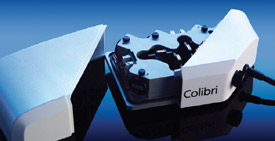
Figure 4 - The modular design of Colibri makes it possible
to easily implement and exploit further developments in LED
technology in the future.
The modules can be easily exchanged by the user,
depending on the experimental design of the day
(Figure 4). The intensity of each module can be
adjusted independently, precisely, and reproducibly
in percentage steps, so that for every fluorescent
dye, the output emitted is precisely what
is needed to achieve the best possible compromise
between the required excitation intensity
and maximum sample protection. The modular
design makes it possible to easily implement and
exploit further developments in LED technology
in the future.
An advantage of LEDs is that they instantly illuminate
at full intensity as soon as electrical current is
applied. Unlike arc lamps that are turned on continuously,
LEDs can be switched on or off instantly
when needed, with no deleterious effects to their life
span. Additionally, with no moving parts, the all-electronic
system is vibration free.
The Colibri system is particularly well-suited
for imaging applications requiring fast switching
between wavelengths. Only about 300 μsec are
needed to switch between the LED modules.
The intensity of every LED module can be adjusted
in percentage steps, enabling equidistant multichannel
images to be easily realized in time-lapse-series.
Instead of adapting the integration times of
the camera to the illumination intensity, LED technology
makes it possible to simply set the illumination
intensity for the required integration time.
The LED illumination intensity is also highly stable
over time, making quantitative analyses easier and
more reliable.
The performance of an imaging system depends not
only on the performance of each
individual component, such as the
light source, but on the sum of all
factors making up such a system.
The system software is a critical,
but often neglected, component.
In order to take full advantage
of the Colibri LED light source
technology, Colibri has been integrated
with the AxioVision imaging
platform (Carl Zeiss).
For live cell imaging, LED technology
is ideally suited to the
fast acquisition framework of
AxioVision,
which forms the backbone
of the Cell Observer HS system
(Carl Zeiss). The integration
of Colibri with AxioVision results
in extremely fast switching times,
with precise control of the illumination
intensity to protect the sample.
Summary
While the intensity of LEDs has
evolved significantly over the past
few years, their intensity is still not
as high as conventional arc lamps.
However, in most live cell imaging
environments, the intensity from
conventional light sources is typically
reduced to minimize phototoxic
effects to cells and tissues.
For applications requiring higher illumination
intensity, or for applications requiring excitation
wavelengths not currently supported by existing
LED technology, the company offers a combination
system that pairs a Colibri with an externally coupled metal halide (HXP) white light source. The
Colibri control panel or the AxioVision software is
used to switch over to and control the shutter of the
HXP 120.
White light sources have been in use for decades,
and much expertise has been developed in the
ways of reducing the problems associated with
peripheral light. Many laboratories have invested
a great deal in filters used to suppress nonspecific
light. In the past, it may have been difficult
to imagine that any other light source method
would ever be viable. Fortunately, a new type
of light source is available. With LED technology,
users can now take advantage of an effective
alternative for live cell imaging, high-speed or
multichannel fluorescence microscopy, and many
other applications.
Ms. Hohman is Product Manager, Light Microscopy, Carl Zeiss
MicroImaging, Inc., One Zeiss Dr., Thornwood, NY 10594,
U.S.A.; tel.: 800-233-2343; e-mail: [email protected].