The attempt to stabilize stationary
phases for use in gas chromatography is
ongoing. This is because lower detection
is increasingly required and, in
order for the detection system to provide
high performance, the risk of contamination
must be minimized. Using
stabilization technology, the breakdown
of stationary phases was reduced, resulting
in low-bleed stationary phases with
tight specifications.
One of the parameters that initiates stationary
phase breakdown is the internal
surface of the GC capillary. Modifying
the surface made it possible to stabilize
stationary phases by a factor of 4–10
lower breakdown. This also resulted in
higher operating temperatures for such
phases as the UltiMetal column (Varian
Inc., Middelburg, The Netherlands) for
simulated distillation applications.
In the present study, arylene-stabilized
5% phenyl/95% methyl polydimethylsiloxane (PDMS) type
phases were tested in a high-temperature GC using fused-silica
columns that were deactivated in a way similar to the
UltiMetal columns. The result is an extremely stable and
low-bleed phase, the VF-5ht (Varian), which is capable of
operating at up to temperatures of 400 °C.
High-temperature capillary columns
Various approaches were taken in the past for performing
high-temperature separations. Columns with an aluminum
outside coating were commercialized 15–20 years ago. In
theory, these columns should have been very temperature
stable and easy to handle. Practical experience with the
aluminum-coated columns revealed, however, that they
became fragile after repeated temperature programming. On
the other hand, isothermal operations performed well. The
difference in thermal expansion coefficient has been the
main cause of this problem.
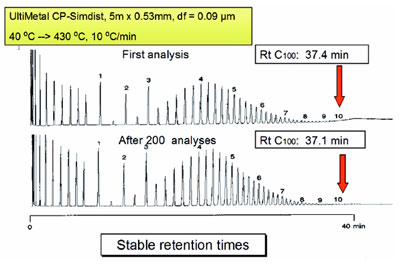
Figure 1 - Retention time stability following long-term, high-temperature exposure using
UltiMetal column coated with 100% PDMS.
Alternative solutions were discovered in 1990,1 when a new
deactivation technology was introduced for the deactivation
of metal capillaries. The resulting columns could be
used at up to very high temperatures. Figure 1 shows an example of such a column for a hydrocarbon sample running
up to C100. The column was used for 200 runs up to
430 °C, and retention time for C100 was altered from 37.4
to 37.1 min. This meant that the majority of the stationary
phase was rendered in the column. This is typical of
UltiMetal Simdist columns, i.e., very low bleed and high
stability. The columns are coated with 100% PDMS-type
phases, providing the best match with boiling-point-type
elution, as required for simulated distillation.
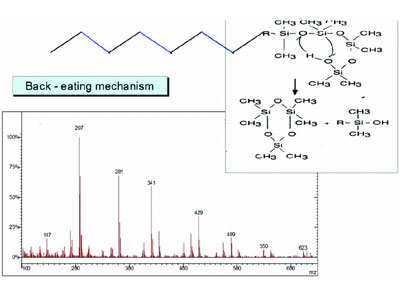
Figure 2 - Breakdown mechanism of siloxane stationary phases: formation
of cyclic degradation products.
Surface stabilization
In order to create a surface that stabilizes stationary
phases, the breakdown mechanism of stationary phases
must be known. Figure 2 depicts the most common mechanism
of siloxane breakdown: A terminal silanol attacks its
own chain and a cyclic (volatile) degradation product is
formed. This volatile degradation product can be detected
by any sensitive detection system. There will be various
ring sizes formed, which can be identified via MS by the
different masses.
There are three mechanisms responsible for the formation of
the terminal silanol group:
- Breakdown initiated by the silanol groups in the stationary phase.
The greater the amount of stationary phase, the more breakdown (bleed)
will be obtained, which is usually why the bleed increases linearly with
the amount of stationary phase in the column.
- Breakdown by the silanols formed upon application. When a
column is operated in a GC, there always will be water molecules
entering the system. This occurs via the carrier gas, leaks, injection,
and the septum. These water molecules will hydrolyze the siloxane chain
and split the siloxane molecule, forming terminal silanol groups. This
again initiates the breakdown and bleed. This can be controlled for
different setups since it depends mainly on the purity of the carrier
gas and how the system is put together and maintained.
- Breakdown initiated by the reactive surface silanol groups. The amount in these groups is determined by the surface and the surface deactivation.
Items 1 and 2 can be easily optimized/minimized using stationary
phase stabilization technologies as well as gas filtration
and thorough leak checking. Item 3 has to be optimized
via the internal column surface.
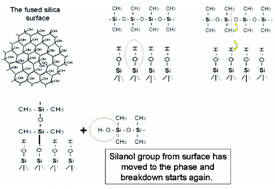
Figure 3 - Surface silanol group transfers to stationary phase, forming
a terminal silanol group.
Normal deactivation using siloxane deactivation reagents
will always leave a number of silanol groups at the surface.
These can react with the stationary phase, forming a surface
bond, and the silanol group will then “jump” to the stationary
phase, as shown in Figure 3. The degradation process
reinitiates, as shown in Figure 2.
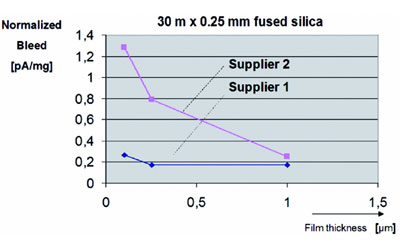
Figure 4 - Normalized bleed (bleed per mg stationary phase) for different
suppliers.
The impact of the surface can be visualized if we calculate the
bleed per milligram of stationary phase (normalized bleeding)
based on the specifications of different suppliers. It is clear
from Figure 4 that by decreasing the film thickness, the bleed
per milligram of stationary phase increases exponentially. This
is due to the impact of the fused-silica surface. Supplier 1 was
more successful at deactivating the reactive surface silanol
groups, resulting in a listing of lower-bleed specifications.
New deactivation of fused-silica surfaces
In addition to the standard siloxane deactivations of surfaces,
a new deactivation was introduced in 1990.2–4 This deactivation
was also applied to fused silica, which resulted in a new
method for extending the temperature stability of stationary
phases. By reducing the level of reactive silanol groups, this
contribution to degradation has been minimized.
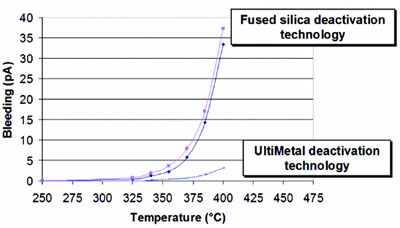
Figure 5 - Impact of column internal surface on stationary phase
degradation.
When coating stabilized stationary phases, there is a significant
improvement in thermal stability. Figure 5 shows a direct comparison of a similar layer of arylene-stabilized
stationary phase, VF-5ms (Varian), coated on a fused-silica,
deactivated surface, and the UltiMetal deactivation
technology. The result is lower degradation of the stationary
phase by a factor of almost 10. The phase stabilized
using this technology is the VF-5ht. These phases can be
used at much higher operating temperatures. Additionally,
when working at lower temperatures, there is hardly any
measurable bleed.
Column lifetime
In high-temperature analysis, column lifetime is often determined
by degradation of the stationary phase. Lower degradation
translates into increased lifetime. Furthermore, retention
times will be more constant, which allows easier
maintenance of integration parameters and calibration.
Although high-temperature phases that use fused silica are
commercially available, they are stabilized mainly by polymer
technologies. The effects of the fused-silica surface
have not been optimized, as shown in Figure 6. A comparison
was made of a 30 m × 0.25 mm fused-silica capillary
coated with a 0.10-μm high-temperature phase. There is a
significant difference in stationary phase degradation. For
high-temperature analysis, the loss of phase usually determines
the lifetime. This is significantly better for the VF-
5ht. When running different sample types, the difference
in column bleed is always seen when the upper temperature
is reached: The background of the VF-5ht is significantly
lower.
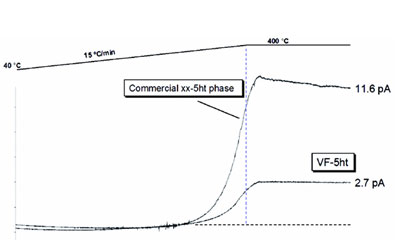
Figure 6 - Comparison of existing high-temperature phases with similar
siloxane composition.
Injection techniques
Most of the high-temperature applications deal with neutral
components. It is important to have a representative
sample on the column; an on-column injection technique
is recommended for this. With on-column injection, the
sample is introduced inside the capillary as a liquid. The
method results in minimal discrimination, since all material
injected onto the column will also elute from the column.
To apply the on-column injection technique, it is
important to have fused silica with a diameter of 0.32 mm in order to allow easy access of the syringe needle into the
capillary. This diameter is standard for the VF-5ht phase.
In addition, the phase is cross-linked and immobilized,
meaning that direct contact with liquids will not change
the column efficiency.
Split systems are also used for high-temperature operations.
If the split system produces a reproducible discrimination,
the technique will also produce acceptable data. Split injection
provides more flexibility with sample types. Column
diameters of 0.25 mm can be used, providing the highest separation
power.
The programmed temperature vaporizer (PTV) is a more
universal technique. It is growing in popularity and is applicable
for high-temperature analysis as well.
Impact of oxygen/water
Oxygen is generally not a problem in GC when analysis temperatures
are low. In some applications, air is used as the carrier
gas at temperatures up to 130 °C. Above 130 °C, the
impact of oxygen increases. When applications are run
higher than 200 °C, oxygen must be removed.
Water can also be a problem at lower temperatures and must
be eliminated. Because water forms terminal silanol groups,
it is the main cause of stationary phase breakdown. As the
reactivity increases with higher temperatures, the high-temperature
applications require ultradry conditions.
Therefore, leaks must be minimized and carrier gas must be
absolutely pure, preferably filtered with special filters before
the GC. Gas Clean filters (Varian) are highly recommended.
With the indicator in the filter, the risk of water
introduction is reduced to a minimum.
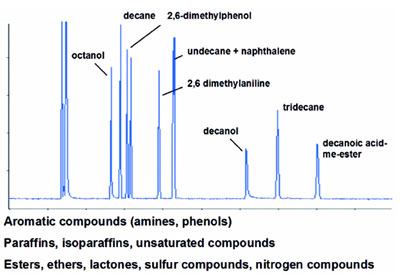
Figure 7 - Different classes of components that can be analyzed using
the high-temperature column.
Loadability/inertness
The high-temperature columns are coated with thin films to
have the lowest possible elution temperatures. The challenge to using thin films is always the loadability and the
inertness of the resulting column. The VF-5ht is a good
compromise. Generally, a wide range of compounds having
different functionalities can be analyzed (see Figure 7).
Highly polar compounds such as alcohols and free acids,
however, will exhibit nonsymmetrical peak shapes. The
majority of high-temperature applications involve neutral or
slightly polar compounds. It is recommended that highly
polar and heavy compounds be derivatized to improve chromatography
and volatility. When using thin-film-coated
columns, there is a constant risk of overloading, and samples
need to be diluted to minimize the overloading effects.
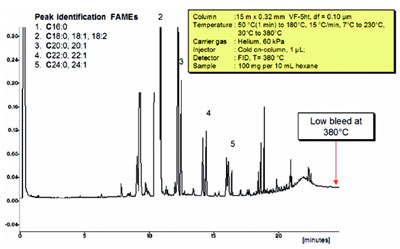
Figure 8 - Analysis of biodiesel according to ASTM D6584.
Applications
The main application area for the VF-5ht phase is for the
separation of high-boiling-point components/mixtures.
ASTM D6584 describes the analysis of biodiesel, which
requires high elution temperatures. Figure 8 shows the separation
of biodiesel using the VF-5ht phase. Up to temperatures
of 380 °C, virtually no bleed can be observed. On-column
injection is used for this application to elute the
heavy boilers with minimal discrimination. In this analysis
in particular, it is important to have good quantification.
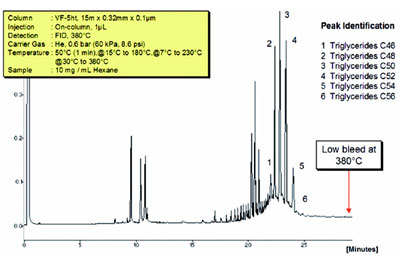
Figure 9 - Triglycerides of palm oil.
The same injection technique was used for the separation of
triglycerides. Separation is by carbon number (see Figure 9).
Since the column has a stabilized polyimide outside coating,
long-term operation at high temperatures will oxidize and
weaken the protective coating. The degree of oxidation
depends on the type of GC, position of the column in the
GC, temperature, and the time exposed. Although the VF-5ht phase on this surface can withstand temperatures of
400 °C, it is recommended that the column be as short as
possible at such high temperatures. High-resolution hydrocarbon
separations can be done using the 0.25-mm-i.d. column,
as demonstrated in Figure 10. The high efficiency of
the columns is also shown. In addition, at higher temperatures,
the VF-5ht phase remains very efficient.
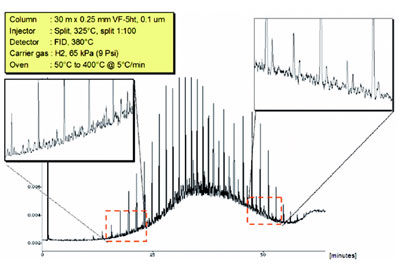
Figure 10 - High-resolution separation of a wax sample.
Conclusion
The VF-5ht stationary phase offers extreme temperature stability,
permitting efficient, high-temperature separations.
The stability of the phase provides reproducible retention
times to allow a reduced number of calibration runs. Column
lifetime is significantly improved. It is important to ensure
that the GC system is absolutely leak free, and carrier gas
must be of high purity in order to gain the full benefit of
these phases for high-temperature separations.
References
- Buyten, J.; Duvekot, C.; Peene, J.; Mussche, P. Eleventh International Symposium on Capillary Chromatography, Monterey, CA, May 14–17, 1990; 91.
- Buyten, J.; Duvekot, C.; Peene, J.; Mussche, P. Int. Chromatogr.
Lab.1990, 2, 5. - De Zeeuw, J. Chrompack News1990, 17(2), 3.
- Schaller, H. Fat Sci. Apr. 1991, 93, 510.
- High Temperature Gas Chromatography. Chrompack, no. 03551, 1990.
The authors are with Varian Inc., Herculesweg 8, 4330 EA Middelburg,
The Netherlands; tel.: +31 118 671279; fax: +31 118 67235; e-mail: [email protected].