Single molecular multianalyte sensors are molecular sensors
that can determine multiple analytes by recognition of the
various analytes based on the difference of the spectral changes
(Figure 1).1,2 To date, many molecular sensors and probes have
been developed that focus on a single analyte. The Ca2+ fluorescent
probe, fura-2,3 is one of the most well-known examples
of such a probe. The authors have also developed highly selective
magnesium fluorescent probes, the KMGs.4–6 Intracellular
imaging using these cation fluorescent probes has been widely
researched. The intracellular signals are based on the correlation
and cross-talk of various messengers; thus, multianalyte
imaging has become increasingly significant.
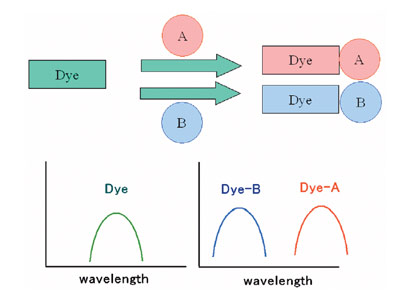
Figure 1 - Single molecular multianalyte sensors/probes.
This paper proposes single molecular multianalyte fluorescent
probes for intracellular multianalyte imaging. By using
single molecular multianalyte fluorescent probes, there is a
minimal invasive effect and quantitative analysis without
localization, metabolism, or photobleaching. Quantitative
analysis can be done with ratiometric calculations.
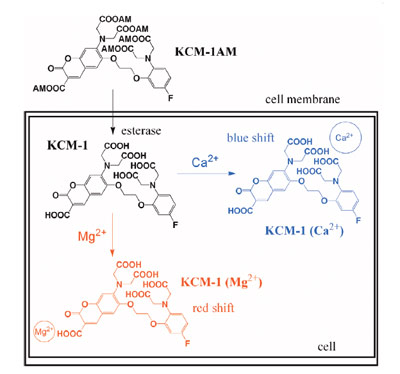
Figure 2 - Schematic diagram of multi-Ca2+/Mg2+ fluorescent probe,
KCM-1, and its intracellular imaging.
Ca2+ is a well-known signal transmitter, and Mg2+ acts as a
cofactor in many situations. The authors focused on the correlations
of the intracellular Ca2+ and Mg2+ with simultaneous
imaging of both cations. The simultaneous imaging of
intracellular Ca2+ and Mg2+ using a novel single molecular
multianalyte sensor, the multi-Ca2+/Mg2+ fluorescent probe,
is shown in Figure 2.
Experimental
Details of the experiment are reported in Ref. 1.
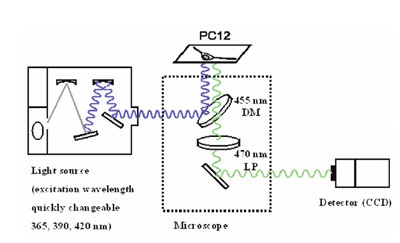
Figure 3 - Excitation wavelength changeable fluorescent microscope
system.
Three images of different excitation wavelengths were
evaluated using an ECLIPSE TE300 inverted microscope (Nikon, Tokyo, Japan) equipped with a 40× S
Fluor objective lens, 505 dichroic mirror, and 535/55
barrier filter (all from Nikon). A 150-W xenon lamp
with a monochromator unit was used for producing three
excitation wavelengths (365, 390, and 420 nm), and the
fluorescence was measured by a HiSCA charge-coupled device (CCD) camera (Hamamatsu Photonics, Hamamatsu,
Japan) (Figure 3).
Cells were incubated with 10 μM KCM-1AM in culture
medium for 30 min at 37 °C and were then washed twice
with a recording solution, followed by further incubation
for 15 min in order to allow complete hydrolysis of the ester
form of the KCM-1AM loaded into the cells.
Discussion
Molecular design of multi-Ca2+/Mg2+
fluorescent probe
The concept of a single molecular multianalyte sensor/probe
has been proposed. Many molecular sensors and probes have
been developed to date, and these molecules are focused
with a high selectivity toward a single analyte. The authors
focused on the optical discrimination of the analyte, in this
case, the molecular design of semiselectivity (insensitivity to
analytes that are not of interest) and discrimination of the
analytes are required, and the evaluated spectra should be
solved by a mathematical solution.
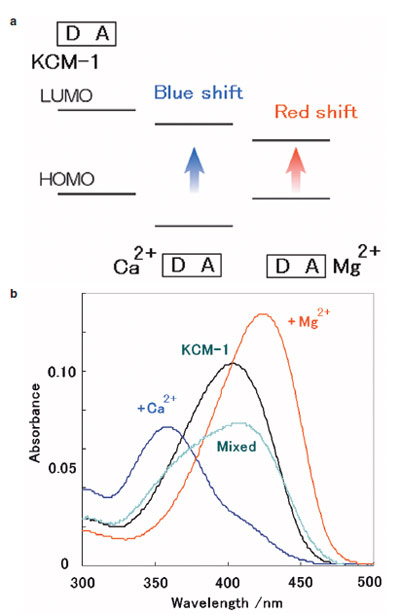
Figure 4 - a) ICT-type mechanism. b) Absorbance spectra of KCM-1 to different Ca2+ and Mg2+ concentrations (measured at pH 7.20, 50
mM 4-(2-hydroxyethyl)piperazine-1-ethanesulfonic acid (HEPES),
130 mM KCl, 20 mM NaCl).
A multi-Ca2+/Mg2+ fluorescent probe had to be designed
to show the different fluorescence (excitation, emission)
change from Ca2+ and Mg2+. Other cations should not bind
to the probe (semiselectivity). The first multi-Ca2+/Mg2+ fluorescent
probe, KCM-1, was designed based on the intramolecular
charge transfer (ICT)-type mechanism. This mechanism
is explained by the change in the energy gap of the dye
molecule, the donor site of the dye having a large coefficient
in highest occupied molecular orbital (HOMO), an acceptor
site with a large coefficient in lowest unoccupied molecular
orbital (LUMO), and a cation interaction stabilizing the
energy level according to electronic interactions (Figure 4a).
It was considered that in the case of a donor–acceptor (D–A) type dye, the cation interaction with the donor site of
the dye induces a larger HOMO stabilization than LUMO,
resulting in a blue shift of the spectra, interaction with the
acceptor site that induces a larger LUMO stabilization than
HOMO, and a red shift (ICT-type mechanism).
It was assumed that the ICT-type mechanism could be used
for the discrimination of multiple analytes; one cation tends
to bind to the donor site with a blue shift of the spectra,
while the other cation binds to the acceptor site with a red
shift. The selective binding sites for Ca2+ and Mg2+ have been
known, i.e., bis-(2-aminophenyl)-ethyleneglycol-tetraacetic
acid (BAPTA) for the Ca2+ binding site, and the charged
beta diketone for the Mg2+ binding site.
In the first multi-Ca2+/Mg2+ fluorescent probe, KCM-1, coumarin
was used as the D–A type fluorophore. BAPTA was used
for the Ca2+ binding site at the donor site of the dye and the
charged beta diketone at the acceptor site, assuming a blue shift
for Ca2+ and a red shift for Mg2+. The dissociation constants of
the BAPTA derivative are typically on the order of 0.1–1 μM
for Ca2+ and 1 mM for Mg2+, while those of the charged beta
diketone are on the order of 10 mM for Ca2+ and 1–10 mM for
Mg2+. A fluorine-substituted BAPTA was chosen as the Ca2+ selective binding site due to its low Mg2+ affinity. KCM-1 was
successfully synthesized by an 11-step synthesis.
Properties of multi-Ca2+/Mg2+
fluorescent probe
Figure 4b shows the absorbance spectra of KCM-1 with Ca2+
and/or Mg2+ under biological conditions. Upon complexation
to Ca2+, KCM-1 shows a 45-nm blue shift in absorbance
and a 5-nm blue shift in the fluorescence spectrum.
For Mg2+, a 21-nm red shift in absorbance and a 5-nm red
shift in fluorescence occurred.
The binding characteristics of fluorescent probes are often
compared based on their dissociation constant, Kd. The
dissociation constants of KCM-1 with Ca2+ or Mg2+ were calculated
to be 14 μM in the case of Kd (Ca2+) and 26 mM for
Kd (Mg2+). While the intracellular Ca2+ concentration is on
the order of 0.1 μM to 1 μM and the Mg2+ concentration is 1
mM, the experimentally determined dissociation constants
indicate relatively low affinities. However, low-affinity indicators
are reportedly useful for the quantification of high
concentrations and transient responses. The properties are
consistent with the molecular design consideration.
Chemical equilibrium and quantification of
multifluorescent probe
Once the difference spectral changes to Ca2+ and Mg2+ have
been achieved, how can the multi-Ca2+/Mg2+ fluorescent probe
work in the presence of both Ca2+ and Mg2+? The optical properties
of KCM-1 were measured for different Ca2+ and Mg2+
concentrations, and the response was compared to the mathematical
simulation that assumes two chemical equilibriums:

Based on the combination of the two equations, the fluorescence
intensity can be solved as follows:

Where K represents the association constant, I is the indicator
(fluorescent probe), ICa2+ and IMg2+ are the indicator–ion complex, and ƒ is a proportionality coefficient for
the individual fluorescent compounds.
This assumption fully agreed with the experimental data for
concentrations less than 10 mM Ca2+ and 10 mM Mg2+ (R>0.999); in the low concentration of Ca2+ and Mg2+, the two
chemical equilibriums Eqs. (1) and (2) were worked out.
While there is much free KCM-1, the binding of the two
cations can be treated independently.
At high Ca2+ and Mg2+ levels, the binding of both analytes to KCM-1 deviated from Eq. (3). However, the effect was small. The binding of one cation to the multiprobe affected
the second binding site by an electron withdrawing effect
and reduced its affinity, which can be considered as an allosteric
effect in an indicator.
For imaging by fluorescent microscopy, Eq. (3) can be solved
using the fluorescent ratio:
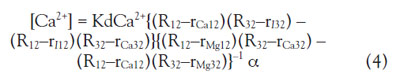

Where Rmn represents the observed ratio of the fluorescence
intensity Fm/Fn (excited at m, n = 1 for 390 nm, 2
for 365 nm, 3 for 420 nm), rImn is the ratio of the free indicator,
rCamn is the ratio for an excess Ca2+ (1 mM), rMgmn
is the ratio for an excess Mg2+ (500 mM), α is the ratio
of the fluorescent intensity at 1 mM Ca2+/free indicator
at 365 nm, and β is the ratio of the fluorescent intensity
at 500 mM Mg2+/free indicator at 365 nm. To solve the
equation, at least three fluorescent intensities at different
excitation/emission wavelengths are required, since
there are two unknown parameters in the system, [Ca2+]
and [Mg2+], and one wavelength for evaluating the fluorescent
ratio (to erase the terms of the concentrations of
the probe)
A microscope system with changeable excitation wavelengths
was constructed (Figure 3). The excitation
light can be quickly changed by an optical chopper at
360/390/420 nm. First, the calibration data and the fluorescent
intensity of the KCM-1, with excess Ca2+ and
Mg2+, should be measured at the three excitation wavelengths.
By measuring the fluorescent images of the PC12
cells imaged at the three wavelengths, the Ca2+ and Mg2+
image can be calculated.
Simultaneous intracellular multianalyte
(Ca2+, Mg2+) imaging
KCM-1 has five carboxyl acids and is highly soluble in water;
therefore, in the case of staining a cell that has a lypophilic
cell membrane, derivatization is required. The acetoxymethyl
ester derivative multi-Ca2+/Mg2+ fluorescent probe,
KCM-1AM, was synthesized. The acetoxymethyl esters
can penetrate the cell membrane and can be cleaved by the
intracellular esterase; thus KCM-1 can be loaded into a cell.
The cytoplasm of PC12 cells was stained effectively with
KCM-1AM and imaged by the fluorescent microscope system.
Eqs. (4) and (5) were solved to evaluate the Ca2+ and
Mg2+ images of KCM-1-loaded PC12 cells.
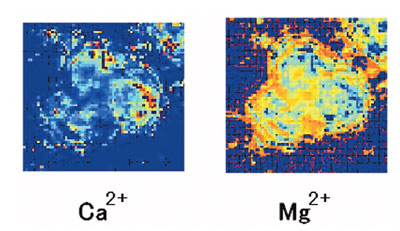
Figure 5 - Simultaneous imaging of intracellular Ca2+ and Mg2+
using the multi-Ca2+/Mg2+ fluorescent probe, KCM-1. PC12 cells
stained by KCM-1AM, imaged by the fluorescent microscope system.
The image is converted. a) Ca2+ image. b) Mg2+ image of PC12 cells
after stimulation by FCCP.
Figure 5 shows the converted Ca2+ and Mg2+ image after
the p-trifluoromethoxyphenyl carbonyl cyanide phenylhydrazone
(FCCP) addition to PC12 cells. By adding mitochondrial
uncoupler, FCCP, to the PC12 cells, increases in
both Ca2+ and Mg2+ were observed based on the release of
mitochondrial Ca2+ and Mg2+.1,4,6 Intracellular Ca2+ and
Mg2+ were successfully imaged by a single multi-Ca2+/Mg2+
fluorescent probe.
Conclusion
A multi-Ca2+/Mg2+ fluorescent probe, KCM-1, was developed.
With KCM-1, intracellular Ca2+ and Mg2+ were
successfully imaged simultaneously. With multianalyte
imaging using a multi-Ca2+/Mg2+ fluorescent probe, the correlation of the intracellular Ca2+ and
Mg2+ in many biological situations,
such as mitochondrial mechanisms,
apoptosis, and energy metabolism,
could be clarified.
The concept of molecular multianalyte
sensors can be extended
to other molecular sensors such
as chromoionophores, green fluorescent
protein (GFP)-based sensors,
and contrast agents. Observations
of multiple signal transmitter
dynamics will clarify the correlation
and cross-talk of biological
signals in the near future.
References
- Komatsu, H.; Miki, T.; Citterio, D.;
Kubota, T.; Shindo, Y.; Kitamura, Y.;
Oka, K.; Suzuki, K. Single molecular
multianalyte (Ca2+, Mg2+) fluorescent
probe and applications to bioimaging.
J. Am. Chem. Soc.2005, 127(31),
10,798–9.
- Komatsu, H.; Citterio, D.; Fujiwara,
Y.; Minamihashi, K.; Araki, Y.; Hagiwara,
M.; Suzuki, K. Single molecular
multianalyte sensor: Jewel Pendant
Ligand. Org. Letters2005, 7(14),
2857–9.
- Grynkiewicz, G.; Poenie, M.; Tsien,
R.Y. J. Biol. Chem.1985, 260(6),
3440–50.
- Komatsu, H.; Iwasawa, N.; Citterio,
D.; Suzuki, Y.; Kubota, T.; Tokuno,
K.; Kitamura, Y.; Oka, K.; Suzuki, K.
Design and synthesis of highly sensitive
and selective fluorescein-derived
magnesium fluorescent probes and
application to intracellular 3D-Mg2+
imaging. J. Am. Chem. Soc.2004,
126(50), 16,353–60.
- Suzuki, Y.; Komatsu, H.; Ikeda, T.;
Saito, N.; Araki, S.; Citterio, D.;
Hisamoto, H.; Kitamura, Y.; Kubota,
T.; Nakagawa, J.; Oka, K.; Suzuki,
K. Design and synthesis of Mg2+-
selective fluoroionophores based on
a coumarin derivative and application
for Mg2+ measurement in a living
cell. Anal. Chem. 2002, 74(6),
1423–8.
- Kubota, T.; Shindo, Y.; Tokuno, K.;
Komatsu, H.; Ogawa, H.; Kudo, S.;
Kitamura, Y.; Suzuki, K.; Oka, K.
Mitochondria are intracellular magnesium
stores: investigation by simultaneous
fluorescent imagings in PC12 cells. Biochim. et Biophys. Molec. Cell
Res.2005, 1744(1), 19–28.
Dr. Komatsu is an Instructor, Dept. of Applied
Chemistry, and Prof. Oka is a Professor, Dept.
of Bioscience and Informatics, Keio University,
3-14-1 Hiyoshi, Kohoku-ku, Yokohama 223-8522, Japan; tel.: +81 45 566 1568; fax:
+81 45 564 5095; e-mail: komatsu@applc.
keio.ac.jp. Prof. Suzuki is a Professor, Dept.
of Applied Chemistry, Keio University, and a
Research Director, Core Research for Evolutional
Science and Technology (CREST), JST
Agency, Saitama, Japan.