Assays that predict passive absorption
of orally administered drugs have
become increasingly important in the
early drug discovery process. A
molecule’s ability to be orally absorbed
is one of the most important aspects in
deciding whether it is a potential lead
candidate. The cell-based assays, like
those using Caco-2 cells, are common
functional models for drug absorption;
however, this labor-intensive and
expensive technique is often pursued
late in the drug discovery process.
Assays described by Kansy1 and Faller2
have addressed these issues by providing
rapid, low-cost, and automation-friendly
methods to measure a compound’s
passive permeability. These in
vitro assays have become an integral
part of early drug discovery because of
their simplicity.3,4 In vitro permeability
assays help predict the potential
bioavailability or in vivo absorption of
a molecule. This paper discusses the
benefits of enhanced throughput,
automation, and robustness of in vitro
permeability assays with progressive
plate technology.
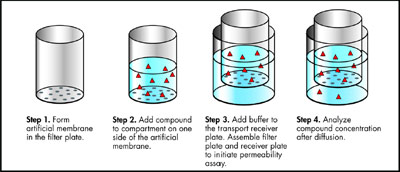
Figure 1 - PAMPA passive diffusion process.
The parallel artificial membrane permeability
assay (PAMPA) is a
noncell-based in vitro technique
used to predict the passive, transcellular
membrane permeability of
potential new pharmaceuticals.
Microplate filters impregnated with
mixtures of phospholipid/organic solvent
fit precisely into discrete
receiver wells to form 96 microdiffusion
chambers. Within the phospholipid
barrier, a concentration gradient
drives the passive diffusion of
molecules, which is the principal
mechanism of drug transport through
biological barriers (see Figure 1).
Data produced by these assays result
in a permeability estimate (effective
permeability, Pe). Fast, flexible, and
cost-effective compared to alternative
cell-based models, PAMPA also
enables the evaluation of different in
vivo absorption models by varying
pH or lipid composition.
Challenges
Traditional products for PAMPA had
limited handling capabilities with
standard laboratory robotics. Automated
assembly introduced trapped air
between the filter and buffer compartments,
reducing diffusion surface area;
receiver well shape limited shaking or
stirring capability; and inconsistent
evaporation necessitated the need for
controlled, humidified environments
due to inconsistent evaporation.
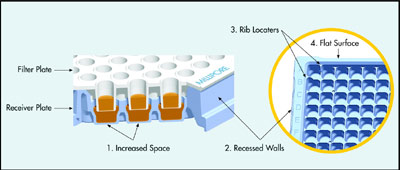
Figure 2 - PAMPA transport receiver design features.
A newly designed transport receiver
plate (Millipore Corp., Danvers, MA)
offers high data quality and throughput
for PAMPA. The molded receiver
plate has such built-in design features
(Figure 2) as increased diffusion space
(1), recessed side walls (2), rib locaters
(3), and a continuous flat top surface
(4) to help overcome many of the
challenges mentioned above.
Experiments
Handling consistency, assay flexibility,
and data reproducibility were
evaluated with standard automation.
Eighteen molded receiver
plates were tested for automated
assembly/disassembly and associated
cross-talk, evaporation, and occurrence
of trapped air. Tests were run
on three consecutive days with
three different automated liquid handlers (Packard® MultiPROBE®
II [PerkinElmer, Boston, MA], Tecan® Genesis™ [Zurich, Switzerland],
and Beckman® BioMek® FX
[Fullerton, CA]). Three machined
Teflon® (DuPont, Wilmington, DE)
receiver plates were used as controls.
Plate performance was evaluated
before, during, and after automated
handling and after overnight
incubation on the robot deck.
Cross-talk and trapped air
Cross-talk (cross-contamination)
between discrete adjacent wells was
measured fluorescently with a Spectrafluor™plate reader (Tecan).
Trapped air was checked visually on a
light box, and well-to-well evaporation
was measured using pathcheck on
the SpectraMax® Plus plate reader
(Molecular Devices, Sunnyvale,
CA). Fluorescent test solution or
buffer (300 μL/well) was dispensed in wells in a criss-cross pattern, and
cross-talk was calculated by dividing
relative fluorescence units (RFU) of
blank wells by RFU of adjacent fluorescent
wells (485-nm excitation/535-nm emission). Molded plates had no
cross-talk and controls averaged 22
wells per plate. Molded plates averaged
one trapped air bubble, while
controls averaged 12 per plate.
Evaporation
Final permeate absorbance is
required to calculate passive transport.
If permeate concentration and
absorbance increase due to evaporative
volume loss, the resulting
calculation leads to artificially
increased permeability rates.
Therefore, minimal and uniform
evaporation are critical for reproducible
and reliable data.
PAMPA incubations at ambient
humidity and temperature were
evaluated for overnight evaporative
volume loss. Based on a 300-μL
starting volume, outer perimeter and
inner well evaporation for the
molded plates was 4.5% ± 0.8% and
4.5% ± 0.6%, respectively (n = 18).
Machined Teflon control loss averaged
8.9% ± 3.8% and 4.6% ± 3.2%
(n = 3), a twofold and fivefold
increase in volume loss and standard
deviation, respectively.
Shaking and stirring
The diffusion rate of molecules
through a phospholipid barrier is
limited by the barrier and/or by
adjacent aqueous boundary layers
(ABL).5 Nonagitated in vitro ABLs
can be 10–100 times greater than
in vivo measurements. When the
ABL is thicker than the phospholipid
barrier, it becomes the rate-limiting
factor for the diffusion of
nonpolar, lipophilic molecules.
Therefore, agitation of the bulk
solution by shaking or stirring can
significantly reduce the ABL,
resulting in more accurate in vitro
permeability predictions.
- Shaking. Six molded plates were
assembled with the BioMek FX liquid
handler and evaluated for
cross-talk after overnight shaking
on the MicroMix5® (DPC, Randolph,
NJ) orbital shaker at
1200–1500 rpm (form 22, amplitude
5). One control plate was
static tested. Fluorescence was
measured with the Spectrafluor plate reader before and after assembly
as well as after overnight shaking.
Molded plates had no cross-talk,
and the control had seven
wells. Molded plates averaged 2.5
trapped air bubbles, and the control
had 22.
- Stirring. Six molded receiver plates
were assembled manually with
super-tumble stir disks (catalogue
no. VP 721F-1, V&P Scientific,
Inc., San Diego, CA) in every
well. Cross-talk was evaluated
after stirring for 1 hr (750 rpm) on
a VP 710C1 rotary tumble stirrer
(V&P Scientific). No cross-talk
was observed.
An integrity test was also performed
for potential stirring-related filter
damage. A lucifer yellow-buffer test
solution (150 μL) was dispensed to
filter plates. After 30 min of stirring
(300 rpm), permeate fluorescence
was measured with a Victor™ spectrofluorometer
(PerkinElmer) at
485/535 nm. Fluorescence of an
equilibrium plate (135 μL/well buffer
+ 65 μL/well test solution) and a
blank buffer plate were required to
calculate integrity. 1-(sample RFU-mean
blank RFU)/(mean equilibrium
RFU-mean blank RFU) = %
integrity. All stirred assemblies had
≥99% filter integrity.
Conclusion
As a result of design enhancements,
PAMPA can be a highly effective
way to characterize and optimize lead
candidates early in the drug discovery
pipeline. Being flexible, fast, low cost,
and automation-friendly,
PAMPA has become the emerging
method to complement cellular based
absorption models.
References
-
Kansy, M.; Senner, F.; Gubernator,
K. Physicochemical high throughput
screening: parallel artificial membrane
permeation assay in the
description of passive absorption
processes. J. Med. Chem. 1998, 41,
1007–10.
- Wohnsland, F.; Faller, B. Highthroughput
permeability pH profile
and high-throughput alkane/water log
P with artificial membranes. J. Med.
Chem. 2001, 44, 923–30.
- Di, L.; Kerns, E. Profiling drug-like
properties in discovery research. Curr.
Opin. Chem. Biol.2003, 7, 402–8.
- Brennan, M.B. Drug discovery (filtering
out failures early in the game).
Chem. Eng. News 2000, 78, 63.
- Kansy, M.; Avdeef, A.; Fischer, H.
Advances in screening for membrane permeability: high resolution PAMPA
for medicinal chemists. Drug Discov.
Today2004, 1, 349–55.
Mr. Kazan is a Biochemical Scientist, Bioscience
Div., Millipore Corp., 17 Cherry Hill Dr.,
Danvers, MA 01923, U.S.A.; tel.: 978-762-
5197; fax: 978-762-5386; e-mail:
[email protected].