The elemental and dynamic range of inductively coupled plasma mass spectrometry (ICP-MS) makes it ideally suited for the analysis of food materials. The ultratrace detection limits of ICP-MS permit the determination of low-level contaminants such as Pb, As, Se, and Hg, while the macro-level nutritional elements such as Ca, Mg, K, and Na can be quantified using the extended dynamic range capability of ICP-MS, which provides nine orders of magnitude. However, there are still a number of challenges to overcome, which makes the routine analysis of foods difficult unless the sample dissolution procedure is well thought out and instrumental conditions are optimized for complex sample matrices.
For example, the wide variety of edible products available means that a highly diverse range of matrices must be brought into solution for ICP-MS analysis. These complex acid-digested matrices, which are a combination of dissolved carbohydrates, fats, and proteins, can pose major problems for any ICP-MS because of the potential for blocking of the interface cones and/or deposition on the quadrupole ion deflector (QID). For this reason, if instrument design does not account for high-matrix samples, long-term stability can be severely compromised.
In addition to signal drift, digested food matrices can also cause major spectral complications. The sample’s organic components, together with macro minerals, can combine with elements present in the digestion acid and/or the plasma argon to form polyatomic interferences. For example, chloride ions (at mass 35) combine with the major argon isotope (mass 40) to produce the argon chloride interference 40Ar35Cl+, which interferes with arsenic at mass 75. Another example is the argon dimer (ArAr+), which forms from the plasma gas and exists at the same masses as the major selenium isotopes. In addition, the major isotope of chromium at mass 52 is overlapped by 40Ar12C, 35Cl17O+, and 35CL16OH+ interferences generated by the sample matrix and the plasma gas. As a result, these kinds of spectral interferences have made the determination of both trace and macro elements in food samples extremely challenging.
To overcome these issues, a NexION® 300X ICP-MS (PerkinElmer, Inc., Shelton, CT) was used for the analysis of various food substances, focusing on toxic and typical essential and macro elements in a group of NIST® (Gaithersburg, MD) standard reference materials (SRMs).
Experimental
Table 1 - Microwave digestion heating program for all six NIST food SRMs
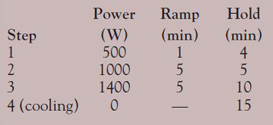
Six different NIST SRM food samples that represent a typical cross-section of the types of foods for human consumption were chosen for the evaluation. The foods included spinach leaves (leafy vegetable), corn bran (grain), wheat flour (grain), bovine muscle (meat), mussel tissue (shellfish), and milk powder (dairy product). The samples were brought into solution with a Multiwave™ 3000 microwave digestion system (Anton Paar GmbH, Graz, Austria). Details of the sample digestion procedure are shown in Table 1.
Sample preparation
Approximately 0.5–0.6 g of each SRM was digested in duplicate with 5 mL of nitric acid (Optima HNO3, Fisher Scientific [Pittsburgh, PA]) and 2 mL of hydrogen peroxide (Optima H2O2) in precleaned Teflon® (DuPont, Wilmington, DE) HF-100 microwave sample vessels (Anton Paar). The filled vessels were placed on a 16-position rotor with an internal p/T sensor positioned in one of the samples to monitor the pressure and temperature inside the sample container. In addition, an external IR sensor provided the temperatures for each individual sample in the tray. The digestion program consisted of 30 min of heating and 15 min of cooling, as shown in Table 1. All the SRM samples were completely dissolved, resulting in clear solutions that were diluted to a final volume of 50 mL with deionized water. No further dilutions were necessary. Gold was added to all solutions at a final concentration of 200 μg/L to stabilize mercury. Preparation blanks, consisting of the acid mixture, were taken through the same microwave digestion program as the samples.
Table 2 - ICP-MS instrumental operating conditions for this application
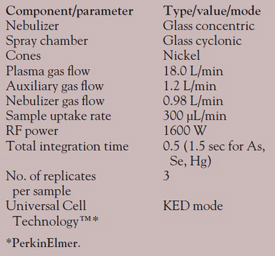
Instrumental conditions
All data in this study were generated under normal operating conditions on a NexION 300X ICP-MS using an autosampler. The instrumental operating conditions are shown in Table 2.
Calibration
Multielement calibration standards, representing all the analytes covered by the six NIST SRMs, were made up from PerkinElmer® Pure single and multielement standards and diluted into 10% HNO3. Gold was added to all solutions at a final concentration of 200 μg/L to stabilize mercury. However, it is important to mention that each food SRM was certified for a slightly different group of elements. For that reason, quantitation was only carried out on the analytes that had reference values. Calibration standard ranges were based on whether the analyte was expected to be a high-level, nutritional element like potassium (K) or sodium (Na), a low/medium-level essential element like manganese (Mn) or iron (Fe), or a trace/ultratrace contaminant such as lead (Pb) or mercury (Hg). Depending on the certificate value of the analytes, five different calibration ranges were made up to cover the complete range of elements being determined. They were:
- High-level nutritional analytes: 0–300 ppm
- Medium-level essential analytes: 0–20 ppm
- Low-level essential analytes: 0–2 ppm
- Trace-level contaminants: 0–200 ppb
- Ultratrace-level contaminants: 0–20 ppb.
Figure 1 shows representative calibration curves for each range.
Figure 1 - Calibration curves for a) 23Na (0–300 ppm), b) 31P (0–20 ppm), c) 54Fe (0–2 ppm), d) 63Cu (0–200 ppb), and e) 78Se (0–20 ppb).
In addition to the analyte elements used for the multielement calibration, the standards, blanks, and samples were also spiked on-line using a mixing tee with a solution of 6Li, Sc, Ge, In, and Tb for internal standardization across the full mass range. Acetic acid was added to the internal standard solution to compensate for residual carbon left over from the sample digestion.