Following an informal collaboration
with Affymax (Palo Alto, CA),
Agilent Technologies (Palo Alto,
CA), and Chem-Space Associates
(Pittsburgh, PA), studying electrospray
ionization 1 (ESI), the technology
invented by Prof. John Fenn,2
a simple technology called induction-based fluidics (IBF) (Nanoliter,
LLC, Henderson, NV)3 was
invented and patented.3 IBF charges
liquids, but without the adverse
electrochemistry inherent in ESI.
IBF has other merits as well. That
said, the charging of liquids has been
around for some time. Prof. Robert
Millikan employed it to determine
the charge on the electron, receiving
the Nobel Prize in 1923,4 and
many others have been charging liquids
for numerous purposes, including
printing, since 1951.5
In IBF, liquids are charged also, and
that action allows the performance of
many simple, useful tasks including
flying nanoliter and microliter quantities
of liquids nontouch to targets
of all types, such as humans, plants,
and animals; microscope slides; multiple-well plates; and scientific instruments.6–12 Through the presentation of
the physics of IBF, it has been shown
that unlike piezoelectric, sound, or any
other technologies that are applied
to transport liquids, IBF technology
employs an electric field that can 1)
kinetically launch drops to targets of all
types, 2) dynamically direct the liquids
in flight (a very desirable, i.e., required,
trait for small volumes of liquids), and
3) count them on arrival. This simple
technology has been called “elegant”
by the director of R&D of a major mass
spectrometry firm,13 and is revisited
here along with new devices and applications
(see Figure 1).

Figure 1 - Nanoliter liquid handling, including 22 nL, 3×, at 60× magnification to a matrix-assisted laser desorption ionization (MALDI) target; 40 nL flown to thumb; nL (varied) flown from a Gilson pipet (Middleton, WI); nL from syringe flown into a multiple-well plate; writing picoliter with pL; ca. 35 nL on dime; 8 channels, 50-nL dispensings; and the parallel LC/MALDI (Nanoliter, LLC), μm-sized droplets directly from LC columns.
Why nanoliters, again?
The nanoliter regime offers a number
of obvious benefits over microliters and milliliters. These include
1) significant savings in expensive
reagents; 2) major reduction in
human exposure to toxic chemicals,
allergens, agents, viruses,
etc.; and 3) greatly reduced waste
disposal costs. Because IBF has a
massive dynamic range (μL to
fL), it has a substantial application
space. It is a useful laboratory
tool that has wide application in,
for example, simple sample dilution;
MALDI biomarkers sample
preparation; drug delivery; drug
discovery; radiochemistry; homeland
security and defense applications;
forensics; the sampling of
human beings; medical diagnostics
sample preparation; and in the
manufacture of unique chemical
and other entities, i.e., electrets.
These charged spheres of reagents
can be manipulated with fields to
effect unique liquid/solid handling
tasks. (These were originally called
nanoliter-sicles.) Finally, IBF
allows nontouch dispensing in the
microliter regime as well, for more
classical assays, and also has interesting
consumer applications.
Applications
Of course there are countless routine
applications of the low-volume
dispensing and aspirating technology,
as given below. Applications
include sample preparation for
thin-layer chromatography (TLC),
or even simple sample dilution or
other sample handling tasks, which
can in fact be highly parallel. This includes the recently patented ability
to morph syringe, peristaltic, and
other pumps into nanoliter dispensers.
Moreover, IBF-based sample
preparation provides the ability to
save precious samples such as expensive
enzymes or evidence in forensic
settings, where disposable containers
can be the dispenser, minimizing
sample transfers and various loss
mechanisms. IBF can dispense, i.e.,
literally fly, nanoliter quantities of
highly viscous, complex liquids such
as glycerin, some glues and slurries,
and even heparinized whole human
blood to targets like microscope
slides, blotting media, and other
targets over short distances (μm)
to longer distances on the order of
meters using simple devices. In certain
settings, IBF can perform nanoliter
or other dispensings from inexpensive,
disposable patent-pending
pipet tips (www. nanoliter.com). IBF
applications demonstrating the wide
and varied interest in low-volume
dispensing are presented in Table 1.
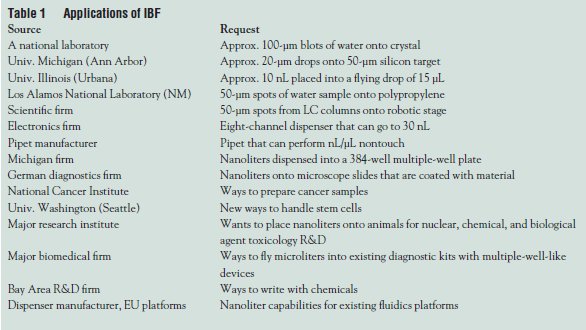
With IBF, one can apply electric
fields of different shape, energy,
polarity, and intensity, and vary
them in countless other ways. Because of this, one can morph
existing traditional devices such
as syringes, pipets, pumps of all
types, LCs, solid-phase extraction (SPE) devices, and other instrument
introduction tools into
new, nontouch dispensing/sample
preparation devices simply by
appending IBF technology to existing
devices. Thus, common tools that are deployed in laboratories
and factories worldwide can take
on useful new attributes that can
facilitate the transport and treatment
of liquids from the macro
to micro to nano world and back,
often extending the dynamic range
of devices by 1, 2, or 3 orders of
magnitude. This property, the ability
to establish “world to nano”
connectivity or to provide a path
from the macro, micro, and nano
world, may prove to be one of the
most useful aspects of IBF. In fact,
one can place liquids onto humans
for drug delivery or sample them using a patent-pending
process
that is nondispersive (see
Figure 2).
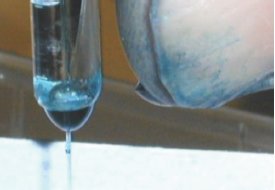
Figure 2 - Liquid being pulled into a cone jet to the target from a human in a nondispersive manner.
Implementation
Recognizing that the technology
was originally introduced
at a very bad time historically,
i.e., September 2001,
one must wonder why the
nanoliter regime has not been
widely adopted given all of its
potential. One possible reason
was the lack of inexpensive,
commercially available tools. To,
in part, remedy this, the nanoliter,
microliter syringe was invented.
This simple device can morph any
common microliter syringe into a
nontouch nanoliter or microliter
dispenser (Nanoliter, LLC). In the
simplest applications, IBF technology
can now deliver cost-effective,
highly accurate, precise, routine,
economical nanoliter liquid handling
to laboratories worldwide.
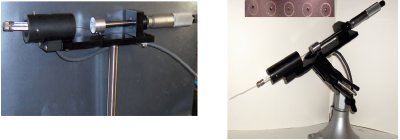
Figure 3 - The nanoliter, microliter syringe with ca. 40-nL dispensings of MALDI (α-cyano-4-hydroxycinnamic acid, CHCA) matrix on a MALDI Applied Biosystems (Foster City, CA) target. Circles are ca. 1 mm. Accurate volumetric and spatial sample placement can improve MALDI results by increasing sample homogeneity and by spatially concentrating analyte in spots the size of the laser beam, as compared to larger volumes of the dried droplet method that results in shooting target locales with no sample, generating prima facie-only noise.
The nanoliter, microliter syringe
(Figure 3) can morph standard microliter
syringes into a nontouch nanoliter
or microliter dispenser once
placed in the chamber. The same
device in 8- or N-channel mode
can place liquids into multiple-well
plates or on surfaces with the volumetric
and spatial accuracy needed
to improve MALDI quantitative and
qualitative analysis when coupled
with the programmable controller
(Nanoliter, LLC). This control can
be seen in a video at www.nanoliter.com
and in Figure 4, which shows the liquids
at the bottom of the multiplewell
plate instead of outside, or on
the sides of the wells, unlike other
technologies that do not dynamically
direct the liquid.
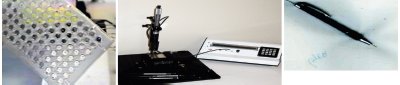
Figure 4 - Nanoliters flown into a multiple-well plate, the patented energy controller, and an IBF spray-based deposition tool based purely on induction.
It is important to realize that IBF
can be used to place samples anywhere,
flying them in simple or
complex trajectories from humans
(not just to them), to instruments,
up to targets (i.e., aspirating
them), down, left, or right, in
a manner completely analogous to
how gas phase ions are flown in
a mass spectrometer as given by
SIMION™ (the software developed
by David Dahl for ion and
electron optics simulation, see www.simion.com). Unlike gas
phase ions in mass spectrometers,
however, IBF-based liquid trajectories
must include other forces.
To understand IBF,5 consider the
physics of a flowing laminar system.
The liquid volume passing through
a tube is given by the Hagen Poiseuille
equation. (IBF does not need
hybrid systems, and the flow can
be purely electrokinetic, but that
is beyond the scope of this paper.)
The volume of fluid (V) that flows
down a small-diameter capillary
tube per unit of time (t) is proportional
to the radius of the tube (r),
the pressure pushing the fluid down
the tube (P), the length of the tube
(l), and the viscosity of the fluid
(η). Note, V is linear in t.
Now, if we grow a drop on a capillary
under these conditions, we can
then charge (q) the drop using an
electric field E to energize it. Upon
charging, the ions rapidly migrate
to the surface of the drop. Now that
the liquid is charged, it can experience
the electrical force (qE)
imparted by induction, similar to
the manner in which gas phase ions
experience the qE force in mass
spectrometers. Since electric fields
can be rapidly toggled on and off
with high accuracy and precision,
the forces on the liquid drops can be changed rapidly and accurately
as well.
Because F is a vector, we can direct
the drop if we shape the field. Thus,
for a charged drop with initial value,
q0, which depends on well-known
solution specifics,
we have a relaxation time, λ,
where λ= (ε0εr/κ), ε0 is the dielectric
constant of free space, εr is the
relative permittivity, κ is the solution
conductivity, t is time, and q
is defined.
Now, a charged liquid drop in an
electric field not only can experience
the qE force, but experiences
different forces as well in the atmosphere
in x, y, and z space, depending
on the specifics of the system, as
stated previously. Using standard,
well-known physics, Newton’s Second
law, we can equate the forces (electric, drag, buoyancy, gravity,
and coulombic) acting on a drop to
those acting in the direction, x, as:
Fx = m (ax) = m (dvx/dt) = Felec + Fdrag + Fbuoy + Fgrav + Fcoul
Force equations can also be written
for the y and z coordinates; therefore,
with accurate model equations
for Fy and Fz, we can actually calculate
the trajectories of the drops
(distances of travel, d) at any time,
t, knowing that Vx = dx/t, Vy = dy/t,
Vz = dx/t, and the initial position of
the drop, and that V2 = Vx2 + Vy2 +
Vz2, but that discussion is beyond the
scope of this paper.
Simple observations of charged liquids
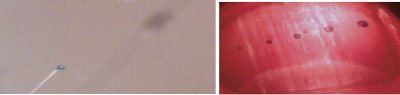
Figure 5 - Visit www.nanoliter.com to see this drop fly up, i.e., aspirate to a thumb; ca. 7.5, 15, 22.5, 45, and 90 nL, estimated using stepper-motor digital readout in this case.
One aspect of IBF is the sometimes
counterintuitive ways that charged
matter behaves. For example, when
a liquid is charged to some value, q,
in a field of strength, E, with gravity
acting in the opposite direction,
the drop can be aspirated, i.e.,
it flies up (www.nanoliter.com). In
fact, it has been known for many
years that one can literally levitate
drops in laboratories. However, with
IBF and this new tool, the technology
can be applied to such activities
as depositing the liquids onto
targets for proteomics biomarkers/cancer disease diagnostics, drug delivery,
placing samples into scientific
instruments, simple dilution, conserving
precious reagents, and countless
other purposes (see Figure 5).