Nanomaterials are important articles of commerce. While a few nanomaterials, such as clays and colloids, are not new, engineered nanoparticles are growing rapidly. Engineered nanoparticles (ENPs) need new assays to monitor the life cycle from creation to recycling or safe disposal. Characterization of nanoparticles is a complex challenge. Key properties include concentration, particle size, particle size distribution, surface charge, surface area, shape, structure, composition, and agglomeration.1 Fortunately, there are many techniques that provide useful measurements of these properties, as listed in Table 1.
Table 1 – Summary of attributes and useful analytical techniques for engineered nanoparticles (source: PerkinElmer2 and industry sources)
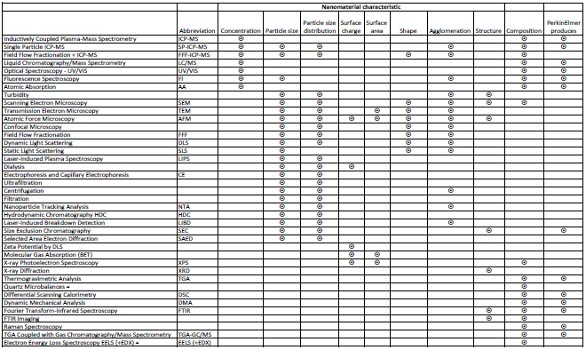
Figure 1 – Chady Stephan.With this background, I was fortunate to interview Dr. Chady Stephan (CS) (Atomic Spectroscopy Product Specialist) (Figure 1) and Andrew Salamon (AS) (Senior Staff Scientist) (Figure 2), members of the PerkinElmer (PE) Analytical Sciences & Laboratory Services nanomaterials characterization team.
RS: PerkinElmer appears to be unique in having a major focus on nanotechnology. How did it start? And what are your vision and goals?
AS: Scientific interest prompted me to investigate this new science and to understand the potential markets for PerkinElmer instruments. I attended the USA Government’s National Nanotechnology Initiative (NNI) meetings,3 developed relationships at many universities, joined the USA ISO Nanotechnologies Technical Committee (TC 229), joined an Industry Nano Consortium, and visited many customers. With this information, I started to educate PE and PE customers about nanotechnology and the characterization of nano-objects. As I proceeded, I found that three nanomaterial markets are very interesting to PerkinElmer: Pharmaceuticals/Medical, Foods, and Environmental.
Figure 2 – Andrew Salamon.My goals are to continue to educate PerkinElmer, PerkinElmer customers, and other groups on nanomaterials, and to listen intently to all nanosituations/issues that PE instruments might be able to solve.
Hopefully, my effort will contribute to developing new analytical techniques that make our lives better.
Particle sizing at the nanoscale level
RS: PE’s new NexION® 300 ICP-MS can count and size single particles. How does it work?
CS: Yes, the NexION 300Q ICP-MS (Figure 3) operated in single-particle mode (SP-ICP-MS) can count and size single particles at the nanoscale level. How does it work? When a particle goes through the plasma, it bursts, creating an ion plume that transits to the detector to create a signal.
Figure 3 – A PerkinElmer product specialist points to the sample intake adapter for the concentric nebulizer of the NexION 300 ICP-MS.SP-ICP-MS science:
- Analyte ions are detected only during an ion plume transit to the detector; otherwise, intensity at the detector is due to background.
- Number of particles counted per second is equal to the particle flux (i.e., every particle entering the plasma is counted).
- The analyte signal intensity is proportional to the ion flux, and the number of ions detected for each plume transit is proportional to the analyte mass in the particle.
- The analyte mass in a particle is proportional to particle size (if the shape is known).
The NexION orthogonal design, with its unique combination of Triple Cone Interface (TCI) and Quadrupole Ion Deflector (QID), provides very low background noise and delivers excellent detection sensitivity.
Other key features of the NexION include: fast data acquisition read speed >3000 pts/sec and scan speed >5000 amu/sec, capability of acquiring data at a 100-μsec dwell time, fast settling time as low as 35 μsec, and a slew speed of 1.6M amu/sec.
RS: What applications are enabled?
CS: Too many to count, and new applications appear frequently. Just look at the six publications that have appeared from the laboratory of Prof. J.F. Ranville of the Colorado School of Mines (Golden, CO) in the first half of 2012.4–10 His group has used (SP) ICP-MS to characterize the polydispersity of metal-containing nanoparticles. This has been aided by field flow fractionation (FFF) to provide sizing.
Carbon nanotubes (CNTs) are made with metal catalysts. Residual levels of metals must be controlled all the way from synthesis to disposal.
Additional applications are listed at www.understandingnano.com/nanoparticles.html.
Nanosilver and single-particle-ICP-MS
RS: Let’s focus on nanosilver. What are your customers doing?
CS: Most of my customers are looking at the fate, transportation, bioavailability, and bioaccumulation of nanosilver.
SP-ICP-MS as an analytical technique is playing an enormous role in advancing our knowledge on the fate, transportation, bioavailability, and bioaccumulation of nanoparticles because of its unique capabilities:
- Excellent detection limits
- Quantification of nanoparticles containing an analyte metal concentration
- Capability to measure the metal mass in individual nanoparticles (correlation with size)
- Ability to track particle dissolution and agglomeration.
RS: How do the results from ICP-MS compare with other sizing techniques such as FFF, light scattering, and ultracentrifugation?
AS: FFF, DLS (dynamic light scattering), SEM (scanning electron microscopy), and TEM (transmission electron microscopy) do not exactly provide the same information. The difference between each technique depends on the sample and the sample prep, plus each technique employs different algorithms to present the data. To qualify the size, the technique used to determine the particle size should be stated.
CS: As Andrew stated, different techniques do not exactly agree, as sample prep, dissolution, aggregation, and instrument algorithms play a big role in data precision.
A good review of SP-ICP-MS and FFF is presented in Ref. 9. In their work, they address the growing need for development of robust analysis methods for ENPs in environmental samples, which typically are below the detection limit of the widely used, existing analysis techniques such as FFF, electron microscopy, or DLS, and where SP-ICP-MS dominates.
SP-ICP-MS is element specific, performs analyses in parts per trillion (ppt) concentration ranges, and is fast. For example, sample analysis time is in seconds using SP-ICP-MS and close to one hour using SdFFF.
Ref. 9 states: “Sizing of these metal-containing ENPs by SP-ICP-MS through determination of instrument transport efficiency and use of a dissolved calibration curve was found to be accurate when compared with results from other commonly used techniques. This illustrates the potential for SP-ICP-MS to be used as a tool for both detection and characterization of polydisperse ENPs in environmental samples.”
Nanoparticles and drug delivery
RS: I’ve read that nanomaterials are being considered for drug delivery. What is the latest?
AS: Drug delivery is a very promising segment with lots of activity. Selectra Biosciences (Watertown, MA) is constructing a virus-like particle that targets influenza virus. Bind Biosciences Inc. (Cambridge, MA) is also developing a targeted nanoparticle to deliver cytotoxic payloads to diseased cells. Cerulean Pharma, Inc. (Cambridge, MA) is in patents with [their] drug candidate CRLX 101. This is described in the “Amazing Results” section on page 7 of the Nanopharmaceuticals White Paper (Nanopharma_009561_01_ WTP-1.pdf) located at www.perkinelmer.com/nano.
Nanolaw
RS: What is the regulatory status of nanomaterials?
AS: There are no regulations for nano-objects in any environment—outdoors, in the workplace, or in our homes.
The California EPA is a leader and trendsetter. I suspect they will have nanolaws in place before the U.S. EPA does.
Analytical applications for engineered nanoparticles
RS: PE is a leading vendor of analytical solutions (Table 1). What other applications are generating the most interest now?
AS: UV-VIS for sizing, TGA (thermogravimetric analysis) for thermal effects on CNTs and NPs. DSC (differential scanning calorimetry) for nanocomposites and heat capacity measurements of nano-objects, DMA (dynamic mechanical analysis) for mechanical properties of nanocomposites, confocal microscopy (3-D imaging), TOF-MS (time of flight-mass spec) for coatings, TGA-GC-MS for coatings on nano-objects.
RS: What about the environmental fate of ENMs?
AS: Some of the leading scientists from government and academic laboratories are investigating ENMs in the environment.
An example is Troy Benn’s and Paul Westerhoff’s (Arizona State University, Tempe) paper on silver nanoparticles into process wastewater treatment sludge, which can be found at: www.sludgenews. org/resources/documents/benn_nanosilver.pdf.
Dr. Jorge Gardea-Torresdey of the University of Texas-El Paso is studying the uptake of nanomaterials by vegetable plants from soil, water, and fertilizers and pesticides. Dr. Arturo Keller at the University of California-Santa Barbara is studying the implications of nanomaterials on aquatic life. Dr. Michele Ostraat at RTI in North Carolina (Chapel Hill) is studying airborne nanomaterials. Dr. E. Sahle-Demessie at the U.S. EPA is studying weathering effects on CNTs in the environment. Dr. Jim Ranville at the Colorado School of Mines is studying the effects of QDs (quantum dots) on the environment.
CS: And I would like to add Dr. Ed Heithmar at U.S. EPA, who is studying the fate of silver nanoparticles in water.10
RS: What about exposure to laboratory staff?
AS: Good point! A recent publication from NIOSH (National Institute for Occupational Safety and Health) describes the risks and provides guidance for working with ENPs in the laboratory: “General Safe Practices for Working with Engineered Nanomaterials in Research Laboratories,” publication #2012-147, www.cdc.gov/niosh/topics/nanotech/pubs.html.
The risks are many and real. For example, nanocopper is a class 3 material (moderately toxic), but 17-micron copper is class 5 (nontoxic). Nanosilver is an anitbactical material used in certain pharmaceuticals to minimize infection.
Nanomaterial exposure modes are inhalation, ingestion, dermal, and ocular, but inhalation is the primary mode, especially for dry samples. Thus, laboratory staff should wear protective devices. In larger quantities, explosion is also a risk since fine particles, especially certain nanometals such as aluminum and zinc, may be more prone to explosion or combustion. Due to their high surface area, they may oxidize quickly.
The publication describes “prevention through design” protocol with the goal of preventing injuries, illness, and fatalities. Figures 19 and 20 provide a quick stratification of risk and risk mitigation strategies.
Analytics in the nanoworld
ICP-MS qualifies as an essential characterization technology for nanomaterials. The ability of PerkinElmer’s NexION 300Q to respond quickly to the material in the plasma’s plume is a key enabling technology that provides scientists with information about the size, dispersity, and composition of nanomaterials, including nanometals. Coupling it to asymmetric flow field flow fractionation (AF4) seems to match the sweet spots of two orthogonal technologies. PerkinElmer deserves credit for recognizing the need for focusing and promoting existing analytics on the new nanoworld.
References
- Hassellhov, M.; Kaegl, R. Analysis and characterization of manufactured nanoparticles in aquatic environments. In: Environmental & Human Health Impacts of Nanotechnology; Lead, J.R.; Smith, E., Eds.; Blackwell Publishing Ltd., Oxford, U.K., 2009, Chapter 6.
- Salamon, A.; Courtney, P. et al. Nanotechnology and Engineered Nanomaterials, A Primer—Frequently Asked Questions, 2010 PerkinElmer Inc., Waltham, MA; www.perkinelmer.com/nano.
- Nanotechnology Initiative (NNI): www.nano.gov/.
- Mitrano, D.; Ranville, J. et al. Field-flow fractionation coupled with ICP-MS for the analysis of engineered nanoparticles in environmental samples. Spectroscopy2012, 27(9), 36–44.
- Ranville, J.; Mitrano, D. et al. An Introduction to Flow Field Flow Fractionation and Coupling to ICP-MS. PerkinElmer, Inc. White Paper; www.perkinelmer.com/CMSResources/Images/44- 132587WTP_FFFCouplingtoICP-MS.pdf.
- Pace, H.E.; Rogers, J. et al. Determining transport efficiency for the purpose of counting and sizing nanoparticles via single particle inductively coupled plasma mass spectrometry. Anal. Chem.2011, 83(24), 9361–9.
- Mitrano, D.M.; Leshner, E.K. et al. Detection of nanoparticulate silver using single particle inductively coupled plasma mass spectrometry. Envir. Toxicol. Chem.2012, 31(1), 115–21.
- Mitrano, D.M.; Barber, A. et al. Silver nanoparticle characterization using single particle ICP-MS (SP-ICP-MS) and asymmetrical flow field flow fractionation ICP-MS. J. Anal. At. Spectrom.2012, 27, 1131–42.
- Reed, R.B.; Higgins, C.P. et al. Overcoming challenges in analysis of polydisperse metal containing nanoparticles by single particle inductively coupled plasma mass spectrometry. J. Anal. At. Spectrom.2012, 27, 1093–1100.
- Heithmar, E.M. Screening Methods for Metal-Containing Nanoparticles in Water, APM 32: U.S. Environmental Protection Agency National Exposure Research Laboratory Environmental Sciences Division Environmental Chemistry Branch, Las Vegas, NV, 2011.
Robert L. Stevenson, Ph.D., is a Consultant and Editor of Separation Science for American Laboratory/Labcompare; e-mail: [email protected].