The genetic information used in the growth and operation of living organisms is contained in DNA, a specific type of nucleic acid molecule. DNA plays a vital role in the process of gene expression.1–10 This incredible molecule has many functions; however, its main role is the long-term storage of genetic information. Nucleic acids are no longer considered inert macromolecules. These molecules are now seen as very dynamic entities that can undertake significant structural changes. An example of such a transitional change involves right-handed double-stranded (ds) B-DNA temporarily adopting the alternative left-handed ds-Z-DNA conformation (Figure 1).8
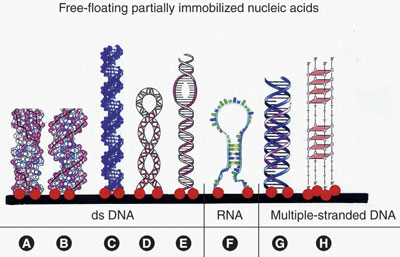
Figure 1 - Transitions in DNA structure. Free-floating partially immobilized DNA and RNA molecules can be immobilized to a microarrays solid support. Eight different molecules are depicted: a) Z-DNA, b) B-DNA, c) B-DNA synthetic polynucleotide, d) negatively supercoiled B-DNA, e) migration of ss-DNA bubble through B-DNA, f) RNA, g) triplex DNA, and h) quadruplex DNA.
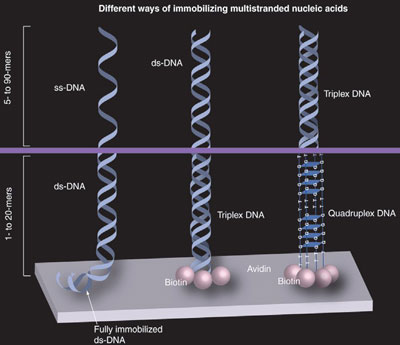
Figure 2 - Different anchoring techniques for multistranded DNAs. Double-stranded DNA can immobilize ss-DNA (first molecule), triplex DNA can anchor ds-DNA (second molecule), and quadruplex DNA can hold triplex DNA (third molecule) to the solid support. The first molecule is immobilized by a small piece of fully anchored ds-DNA. The second and third molecules are anchored by a biotin–avidin connection. The horizontal line represents the transitional points between the different DNA structures.
Gene expression is controlled by many factors, such as the DNA base pairs, DNA-binding proteins, RNA-binding proteins, and chemicals, and by structural changes that occur in DNA.2,5–10 The structural polymorphisms of many DNAs are dependent on the immediate environment, viz., nucleic acid-binding proteins, polyamines, and ionic strength. Alternative DNA structures, such as Z-DNA, can be induced by DNA supercoiling.8 Nucleic acids can also assume other alternative structures, such as anisomorphic DNA, Holliday intermediates, parallel-stranded DNA, mispaired DNA, DNA–RNA hybrid sequences, B-DNA/Z-DNA junctions, cruciform configurations, catenanes, hairpin nucleic acids, triple-stranded DNA, four-stranded DNA, and five-stranded DNA (Figures 1 and 2).2,6–8 As a result, the study of DNA and RNA sequences, distribution, and content alone does not provide scientists with an adequate understanding of the factors that control the expression of genes. To more completely characterize the expression of genes and locate new DNA and RNA target sites for drugs, scientists must focus more on DNA and RNA sequences in genes that have the possibility of assuming structural transitions, and examine multistranded and alternative nucleic acid structures.2,5,6,9,10
Conventional DNA and RNA microarrays
DNA microarrays are a new and extremely potent means of characterizing genomes and proteomes.2,6 Conventional DNA microarrays are used to study location analysis of DNA and to characterize changes in gene expression. Conventional microarrays are based on primary structure only, namely, base pairs of single-stranded (ss) DNA (i.e., DNA probe) that hybridizes with a target element. They allow for in-depth analysis of gene expression. However, this only tells a part of the genomic/proteomic story. In comparison, the new microarrays employ nondenatured, intact DNAs or RNAs. This results in a three-dimensional structure, similar to what may be found in vivo. Therefore, the microarrays maintain unaltered nucleic acids for isolation, characterization, and/or experimentation. With the DNA and RNA microarrays, it is possible to study the lingering alternative nucleic acids that also regulate life.2,5,6,9,10
The new arrays go further than conventional gene expression analysis systems; they sanction the characterization of unaltered, intact, nondenatured, alternative, conventional, and multistranded nucleic acids, and nucleic acid–protein binding complexes. They also allow interactions with other DNAs, RNAs, proteins, pharmaceuticals, and/or environmental situations. The microarray technology will enhance cell biology, molecular biology, biochemistry, pathology, synthesis and sequencing of DNA or RNA, transcription–translation studies, acceleration of the drug discovery process, and discovery of new gene–drug and protein–drug target sites.
Next generation of DNA and RNA microarrays: alternative and multistranded nucleic acids and nucleic acid structural transitions
In the process described here,2,6,9,10 the arrays anchor unaltered, intact, nondenatured DNA and RNA employing new substrate surfaces. The substrate surface enhances immobilization of DNA and RNA molecules, therefore allowing for the extended characterization of DNA and RNA structural transitions and testing of pharmaceuticals for their capacity to interact with nucleic acids under many different environments.
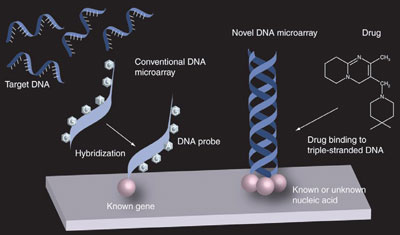
Figure 3 - Comparison of conventional DNA microarrays with the multistranded nucleic acid microarrays. Conventional DNA microarrays (i.e., hybridization) differ from the microarrays described in this article (i.e., a drug binding to triplex DNA).
Standard microarrays have become one of the most powerful tools used by researchers to characterize gene expression. Figure 3 shows one of the differences between conventional microarrays (i.e., hybridization) and the DNA and RNA microarrays discussed in this article (i.e., examination of new drug candidates). Most notably, unlike conventional DNA microarrays that anchor ss-DNA, these DNA and RNA microarrays immobilize intact nucleic acids. The nondenatured nucleic acids can be immobilized onto the solid support either directly (i.e., presynthesized) or can gradually be synthesized. The microarrays also permit the immobilization of many other types of nucleic acids: ds-B-DNA, ds-A-DNA, ds-C-DNA, ds-D-DNA, ds-Z-DNA, A-RNA, ds-RNA, cruciform nucleic acids, DNA–RNA hybrids, parallel-stranded DNAs, triplex DNA, quadruplex DNA, pentaplex DNA, peptide nucleic acid (PNA), and locked nucleic acid (LNA). The technique will enable the development of many new types of nucleic acid microarrays (e.g., Z-DNA, quadruplex DNA, and cruciform DNA), and will become an important tool for experimenting with nucleic acids and isolating drugs for biopharmaceutical research.2,6,9,10
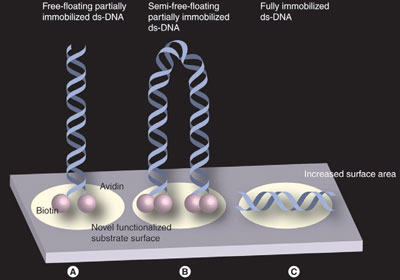
Figure 4 - Three techniques of anchoring DNA: a) Free-floating partially immobilized ds-DNA is anchored at one end by a biotin–avidin connection. b) Semifree floating partially immobilized ds-DNA is anchored by both ends using two biotin–avidin connections. c) Fully immobilized ds-DNA.
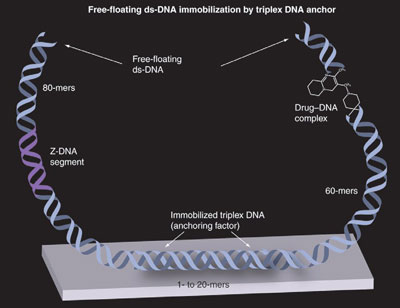
Figure 5 - Anchoring of two ds-DNAs (i.e., experimental-target sites) by a triplex DNA anchoring procedure. Two separate free-floating partially immobilized ds-DNAs are immobilized to the solid support by a triplex DNA molecule. The 60-mers of ds-B-DNA has a drug binding to it (i.e., characterization of pharmaceutical). The 80-mers segment has a sequence of DNA with the potential to convert to left-handed Z-DNA.
Molecules can be immobilized using many different approaches, i.e., free-floating partially anchored (Figures 1–5), semifree-floating partially immobilized (Figure 4b), and fully anchored DNAs or RNAs (Figure 4c). The alternative and multistranded nucleic acid molecules can be immobilized via many different noncovalent attachment mechanism protocols.2,6,9,10 Covalent immobilization can be used to effectively anchor the multistranded nucleic acids. An example of this process can be seen with thiol-modified DNA (mercaptopropylsilane), amino-terminal DNA (epoxide-coated slide), disulfide-modified DNA, phosphorylated DNA, and carboxylated DNA.
The alternative DNA and RNA molecules can also be covalently immobilized to unmodified substrate surfaces (e.g., silanized DNA). Construction parameters (i.e., noncovalent and/or covalent) of alternative and multistranded DNA and RNA microarrays depend on many different factors, including width and length of DNAs, structural transitions of DNAs, base pairs, specific and nonspecific interaction of DNAs and RNAs to the substrate surface, inkjetting or spotting of DNA, mechanical or in situ synthesis deposition of premade DNAs and RNAs, and end-user procedures.