Over the last decade, monolith technology has progressed from a promising approach conceived by Fréchet, Švec, Huber, and others1–6 to a well-developed separation tool. Its role in HPLC has been reviewed.7 Monolith technology competes with bead-based technologies by creating new approaches to bioapplications.8–13 The current interest in monolith technology is based on its ability to achieve desired functionalities14 with high-capacity, high-speed separations and a limited compromise in resolution. Organic monoliths are capable of providing rapid separations of biomolecules because the separation mechanism is driven primarily by convective rather than diffusive mass transfer. Band broadening is reduced at high flow rates. High mass loading capacities also make monoliths well suited for the first-dimension separation of less abundant target proteins in crude biological samples. Automation of 2-D LC with fraction collection is widely accepted for separation and identification in the biotechnology industry.15,16
Polymer monoliths produced in polyetherether ketone (PEEK™, Victrex PLC, Lancashire, U.K.) housings provide very good pH stability, unlike silica monoliths or those prepared in fused-silica capillaries. High pH stability is often required for sterilization. In addition, polymer monoliths exhibit broad solvent compatibility and pressure robustness.
In the study described here, various protein separations were performed with 1-mm-i.d. columns using different ion exchange and reversed-phase functionalities. The examples provided include the improved sensitivity of 1-mm columns compared to larger-i.d. formats, dynamic loading, column ruggedness, run time stability, pH stability, and lot-to-lot reproducibility.
Materials and methods
Chemicals
Proteins and all other analytical-grade chemicals were obtained from Sigma Aldrich (St. Louis, MO). E. coli protein was from Bio-Rad (Hercules, CA).
HPLC instrumentation
Reversed-phase chromatography was carried out using a P680 HPG gradient pump, UVD 340 detector, WPS 3000 TSL autosampler, and TCC-100 thermostated column compartment. Ion exchange chromatography was performed with an inert PEEK ICS 3000 DP gradient pump, VWD 3000 detector, AS autosampler, and TCC-100 thermostated column compartment. A microflow cell (2.5 μL) was used with UV detectors. The narrow-bore tubing (0.003 in. i.d.) was used between the pumps and the detector to reduce the delay volume. All chromatography components were from Dionex Corp. (Sunnyvale, CA). Chromatographic equipment control and data acquisition were via Chromeleon® chromatography data system software from Dionex.
Monolithic HPLC columns
The following columns were used: ProSwift® WCX-1S (1 × 50 mm), ProSwift WAX-1S (1 × 50 mm; 4.6 × 50 mm), ProSwift SAX-1S (1 × 50 mm), and ProSwift RP-4H (1 × 250 mm).
Eluents
- ProSwift WCX-1S—eluent A: 10 mM sodium phosphate (pH 7.6); eluent B: 1 M sodium chloride in eluent A
- ProSwift WAX-1S and ProSwift SAX-1S—eluent A: 10 mM Tris (pH 7.6); eluent B: 1 M NaCl in eluent A
- ProSwift RP-4H—eluent A: deionized water: acetonitrile (95:5, v/v) plus 0.1% (vol) trifluoroacetic acid (TFA); eluent B: acetonitrile:deionized water (95:5 v/v) plus 0.1% (vol) TFA.
Dynamic capacity of monolithic weak cation exchange (WCX) columns
Increased amounts of protein mixture (1–24 μL of 1 mg/mL each) containing ribonuclease A, cytochrome C, and lysozyme were injected on the ProSwift WCX 1 × 50 mm column. Sample load at 50% increase in peak width at half height (PWHH) is considered a measure of dynamic capacity of the column for that specific protein.
Ruggedness and run time stability test
Ruggedness was evaluated by means of run time stability for ProSwift monolithic ion exchange and reversed-phased (RP) columns. Specific analytes were separated and their retention times were recorded up to 250 runs for weak cation exchangers and about 400 runs for anion exchangers and reversed-phase functionalities. For chromatography on the WCX-1S 1 × 50 mm, WAX- 1S 1 × 50 mm, SAX-1S 1 × 50 mm, and RP-4H 1 × 250 mm columns, the proteins lysozyme, ovalbumin, trypsin inhibitor, and myoglobin were used, respectively. The protein was injected intermittently between the second to tenth blank runs. Different flow rates and gradients were used depending on the column under test.
Results and discussion
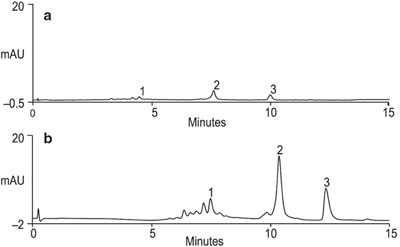
Figure 1 - Separation of a protein mixture on ProSwift WAX-1S columns. Comparison of mass sensitivity of a 1-mm-i.d. column with a 4.6-mm-i.d. column format. ProSwift WAX-1S (4.6 × 50 mm [a]) (1 × 50 mm [b]) tested at 2.1 mL/min (a) and 0.2 mL/min (b), respectively. Sample: ovalbumin (1 mg/mL), trypsin inhibitor (1 mg/mL), and insulin chain A (0.25 mg/mL); injection volume: 1.3 μL; gradient: 5–55%B in 13 min; detection: UV at 280 nm.
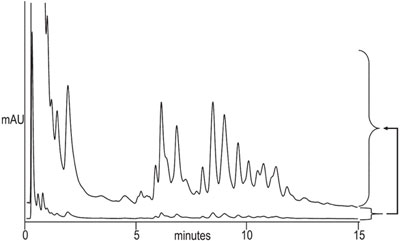
Figure 2 - Separation of E. coli proteins on ProSwift WAX- 1S (1 × 50 mm) column. Sample: E. coli proteins (2.7 mg/mL); injection volume: 1.3 μL; gradient: 5–55%B in 13 min; flow rate: 0.2 mL/min; detection: UV at 280 nm. The upper trace is the enlarged version of the bottom trace.
ProSwift monolithic columns are porous polymer-based structures that offer significant advantages such as high resolution and high loading capacity. These characteristics support versatile performance in a wide range of biomolecule separations.
When comparing the separation of a standard protein mixture on a ProSwift WAX-1S column in two different formats using equivalent separation conditions (Figure 1), an increase in mass sensitivity is observed. The results show improved sensitivity with the 1 × 50 mm format versus the 4.6 × 50 format. A 1-mm column format is best when the sample amount is limited and higher sensitivity is desired. It is especially useful when analyzing cell lysates for low-copy-number proteins. Since the majority of the proteins found in the E. coli sample (Figure 2) are anionic at pH 7.6, they can be separated using the anion exchanger.
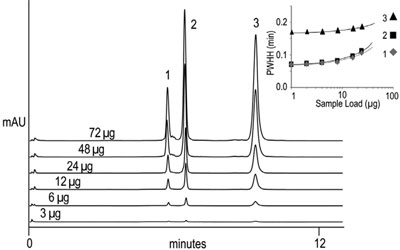
Figure 3 - Dynamic protein loading capacity on ProSwift WCX 1 × 50 mm column. Samples: 1) ribonuclease A, 2) cytochrome C, and 3) lysozyme (1 mg/mL each); injection volume: 1, 2, 4, 8, 16, or 24 μL. Inset: plot showing the increase in PWHH in minutes vs sample load. Gradient: 0–85%B in 7.5 min; flow rate: 0.2 mL/ min; detection: UV at 280 nm.
The dynamic protein loading of a weak cation exchange column (ProSwift WCX-1S) is shown in Figure 3. The separation of protein variants is not compromised with increased sample loading (up to 72 μg total protein on column), suggesting the high loading capacity and separation power of these monolithic columns. The loading plot (inset, Figure 3) illustrates that the peak width is only slightly affected at this loading. When loading a mixture of proteins, the PWHH of ribonuclease A and cytochrome c increased by 50% at a loading of 24 μg, whereas lysozyme PWHH did not. Proteins can usually be loaded well over the amount of the 50% PWHH limit as long as desirable resolution of the target protein(s) is achieved.